Harnessing the power of RADseq for ecological and evolutionary genomics - PubMed
Review
Harnessing the power of RADseq for ecological and evolutionary genomics
Kimberly R Andrews et al. Nat Rev Genet. 2016 Feb.
Abstract
High-throughput techniques based on restriction site-associated DNA sequencing (RADseq) are enabling the low-cost discovery and genotyping of thousands of genetic markers for any species, including non-model organisms, which is revolutionizing ecological, evolutionary and conservation genetics. Technical differences among these methods lead to important considerations for all steps of genomics studies, from the specific scientific questions that can be addressed, and the costs of library preparation and sequencing, to the types of bias and error inherent in the resulting data. In this Review, we provide a comprehensive discussion of RADseq methods to aid researchers in choosing among the many different approaches and avoiding erroneous scientific conclusions from RADseq data, a problem that has plagued other genetic marker types in the past.
Figures
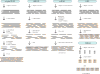
Step-by-step illustration of five RADseq library prep protocols. All protocols begin by digesting relatively high quality genomic DNA with one or more restriction enzymes. For most protocols, the sequencing adapters (oligos) are added in two stages, with one set of oligos added during a ligation step early in the protocol, and a second set of oligos incorporated during a final PCR step. The second set of oligos extends the length of the total fragment to produce the entire Illumina adapter sequences. In contrast, the original RADseq adds adapters in three stages. For Illumina sequencing, the adapters on either end of each DNA fragment must differ, and therefore some protocols (e.g. original RADseq, ddRAD, ezRAD) use “Y-adapters” that are structured to ensure that only fragments with different adapters on either end are PCR-amplified (illustrated here as Y-shaped adapters). Other protocols (e.g. GBS) simply rely on the fact that fragments without the correct adaptors will not be sequenced. To generate fragments of an ideal length for sequencing, most methods use common-cutter enzymes (e.g. 4–6bp cutters) to generate a wide range of fragment sizes, followed by a direct size selection (gel-cutting or magnetic beads, e.g. ddRAD, ezRAD) or an indirect size selection (as a consequence of PCR amplification or sequencing efficiency, e.g. GBS). In contrast, the original RADseq uses a mechanical shearing step to produce fragments of an appropriate size, and incorporates a size selection step only to increase Illumina sequencing efficiency and remove adapter dimers. 2bRAD uses IIB restriction enzymes to produce small fragments of equal size across all loci (33–36bp).
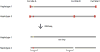
Sources of error and bias in RADseq data. (a) Example of allele dropout for a RADseq protocol that uses size selection to reduce the number of loci to be sequenced. Gray lines represent chromosomes within one individual, red squares represent restriction cut sites, colored squares represent heterozygous SNPs, and brackets represent genomic regions that are sequenced. Mutation in Restriction Cut Site B for Haplotype 1 makes the post-digestion fragment containing the SNP too long to be retained during size selection for Haplotype 1, eliminating the possibility of sequencing of any loci on that fragment, and causing the individual to appear homozygous at the heterozygous SNP. (b) See Figure 1 of Andrews et al. 2014. Example of fragments produced after PCR for one heterozygous locus for different RADseq protocols, and the reads retained after bioinformatic analyses. PCR duplicates are shown with the same symbol (circle, square, asterisk or triangle) as the parent fragment from the original template DNA. By chance, some alleles will amplify more than others during PCR. For all protocols, PCR duplicates will be identical in sequence composition and length to the original template molecule. For the original RADseq, this feature (i.e., identical length) can be used to identify and remove PCR duplicates bioinformatically, because original template molecules for a given locus will not be identical in length. For alternative RADseq methods, this feature cannot be used to identify PCR duplicates, because all original template molecules for a given locus are identical in length. High frequencies of PCR duplicates can cause heterozygotes to appear as homozygotes or can cause PCR errors to appear as true diversity.
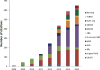
Numbers of articles citing the original papers describing each RADseq protocol over time. Data for 2015 are extrapolated using numbers of articles cited from January through September 2015. Protocols are arranged by order of first appearance in the literature. Data generated using Web of Science.
Similar articles
-
Trade-offs and utility of alternative RADseq methods: reply to Puritz et al.
Andrews KR, Hohenlohe PA, Miller MR, Hand BK, Seeb JE, Luikart G. Andrews KR, et al. Mol Ecol. 2014 Dec;23(24):5943-6. doi: 10.1111/mec.12964. Mol Ecol. 2014. PMID: 25319129
-
Population genomic analysis of model and nonmodel organisms using sequenced RAD tags.
Hohenlohe PA, Catchen J, Cresko WA. Hohenlohe PA, et al. Methods Mol Biol. 2012;888:235-60. doi: 10.1007/978-1-61779-870-2_14. Methods Mol Biol. 2012. PMID: 22665285
-
Schmidt DA, Campbell NR, Govindarajulu P, Larsen KW, Russello MA. Schmidt DA, et al. Mol Ecol Resour. 2020 Jan;20(1):114-124. doi: 10.1111/1755-0998.13090. Epub 2019 Sep 24. Mol Ecol Resour. 2020. PMID: 31483931
-
Genomic Methods Take the Plunge: Recent Advances in High-Throughput Sequencing of Marine Mammals.
Cammen KM, Andrews KR, Carroll EL, Foote AD, Humble E, Khudyakov JI, Louis M, McGowen MR, Olsen MT, Van Cise AM. Cammen KM, et al. J Hered. 2016 Nov;107(6):481-95. doi: 10.1093/jhered/esw044. Epub 2016 Aug 10. J Hered. 2016. PMID: 27511190 Review.
-
Targeted capture in evolutionary and ecological genomics.
Jones MR, Good JM. Jones MR, et al. Mol Ecol. 2016 Jan;25(1):185-202. doi: 10.1111/mec.13304. Epub 2015 Jul 30. Mol Ecol. 2016. PMID: 26137993 Free PMC article. Review.
Cited by
-
Perry KD, Keller MA, Baxter SW. Perry KD, et al. Sci Rep. 2020 Jul 21;10(1):12047. doi: 10.1038/s41598-020-68140-w. Sci Rep. 2020. PMID: 32694639 Free PMC article.
-
Ma J, Cao Y, Wang Y, Ding Y. Ma J, et al. Front Plant Sci. 2022 Nov 11;13:972791. doi: 10.3389/fpls.2022.972791. eCollection 2022. Front Plant Sci. 2022. PMID: 36438102 Free PMC article.
-
Yang GQ, Chen YM, Wang JP, Guo C, Zhao L, Wang XY, Guo Y, Li L, Li DZ, Guo ZH. Yang GQ, et al. Plant Methods. 2016 Aug 4;12:39. doi: 10.1186/s13007-016-0139-1. eCollection 2016. Plant Methods. 2016. PMID: 27493679 Free PMC article.
-
Landis JB, Miller CM, Broz AK, Bennett AA, Carrasquilla-Garcia N, Cook DR, Last RL, Bedinger PA, Moghe GD. Landis JB, et al. Mol Biol Evol. 2021 Jul 29;38(8):3202-3219. doi: 10.1093/molbev/msab092. Mol Biol Evol. 2021. PMID: 33822137 Free PMC article.
-
Bergamo LW, Silva-Brandão KL, Vicentini R, Fresia P, Azeredo-Espin AML. Bergamo LW, et al. Insects. 2020 Aug 16;11(8):539. doi: 10.3390/insects11080539. Insects. 2020. PMID: 32824385 Free PMC article.
References
Publication types
MeSH terms
Grants and funding
LinkOut - more resources
Full Text Sources
Other Literature Sources
Miscellaneous