A new time tree reveals Earth history's imprint on the evolution of modern birds - PubMed
- ️Thu Jan 01 2015
A new time tree reveals Earth history's imprint on the evolution of modern birds
Santiago Claramunt et al. Sci Adv. 2015.
Abstract
Determining the timing of diversification of modern birds has been difficult. We combined DNA sequences of clock-like genes for most avian families with 130 fossil birds to generate a new time tree for Neornithes and investigated their biogeographic and diversification dynamics. We found that the most recent common ancestor of modern birds inhabited South America around 95 million years ago, but it was not until the Cretaceous-Paleogene transition (66 million years ago) that Neornithes began to diversify rapidly around the world. Birds used two main dispersion routes: reaching the Old World through North America, and reaching Australia and Zealandia through Antarctica. Net diversification rates increased during periods of global cooling, suggesting that fragmentation of tropical biomes stimulated speciation. Thus, we found pervasive evidence that avian evolution has been influenced by plate tectonics and environmental change, two basic features of Earth's dynamics.
Keywords: K-Pg mass extinction; avian evolution; divergence times; diversification rates; global biogeography.
Figures
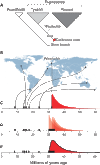
(A) The fossil Wieslochia weissi from the Oligocene of Germany shows an apomorphy of the suborder Tyranni: a well-developed tuberculum ligamenti collateralis ventralis. Therefore, Wieslochia sets an absolute minimum age for the time of origin (stem age) of the Tyranni, which is the same as the crown age of Eupasseres. The oldest fossil of the suborder Passeri would also set a minimum age for Eupasseres, but it is younger than Wieslochia. Note that fossils that only show an apomorphy of Eupasseres may be in the stem branch; for this reason, they are not informative regarding the minimum age of crown Eupasseres. (B) The first record of Eupasseres (Tyranni or Passeri) in other continents completes a set of fossil occurrences of ages t1…tn (see data set 1 for details). (C) This set of ages does not depart significantly from a uniform distribution (Kolmogorov-Smirnov test, D = 0.25, P = 0.7). Therefore, the likelihood function for the upper bound (θ) of a uniform distribution [1/(θ)n for θ > tn] is used to generate a distribution for the true age of Eupasseres. (D) However, the oldest fossil does not have a precise age estimate but was assigned to a geological time interval that spans several million years (the same is true for other fossils in the set, but their ages do not influence the likelihood). Therefore, pseudosamples of tn are generated by sampling uniformly from the time interval to which the oldest fossil was assigned and used for generating multiple distributions of clade age. (E) Averaging these pseudoreplicated distributions results in a final distribution, which is then modeled with a standard probability density function that mimics its shape: a log-normal distribution, a log mean of 1.7, and a log SD of 0.8. This standard distribution is used as clade age prior in Bayesian divergence time estimation.
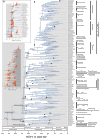
Maximum clade credibility (MCC) trees from a Bayesian divergence time estimation using calibration priors inferred from the fossil record. (A) MCC tree from the analysis of 124,196 bases from the first and second codon positions of 1156 clock-like genes from Jarvis et al. (3) and 10 calibration priors. (B) MCC tree from the analysis of 4092 bases of the recombination-activating genes for 230 species and 24 calibration priors. Black diamonds are calibration nodes, black dots are clades that were constrained to match relationships supported by recent multilocus and genomic analyses (3, 28), red density distributions are clade age prior probabilities derived empirically from a quantitative analysis of the fossil record of clades, and blue bars represent 95% highest posterior densities for node age from the posterior distribution. Median ages are indicated for large clades mentioned in the text (blue dots).
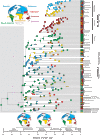
Fitch parsimony optimizations of ancestral geographic regions are shown at the nodes. Multiple regions at a node represent alternative, equally parsimonious optimizations. The tree is the maximum clade credibility tree of a Bayesian analysis of recombination-activating genes for 230 species and 24 calibration priors and with the addition a posteriori of 25 fossil taxa that represent Holarctic distributions for clades now restricted to the tropics. Distributions at the tips are those of the clades they represent. Schematic representations of global paleogeography at the K-Pg transition, the middle Eocene, and the middle Miocene are shown together with major postulated interregional connections as inferred from paleogeographic evidence and biogeographic analysis. Higher-level taxa are indicated on the right (see fig. S2 for species names).
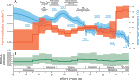
(A) Red: Net lineage diversification rate (speciation-extinction) estimated for 5-Ma intervals using birth-death shifts models (120) (lines are medians of 500 estimates from a sample of the posterior distribution of trees from the Bayesian time-tree analysis; light boxes around medians are interquartile ranges). Blue: Deep-ocean temperatures estimated from a global compilation of benthic foraminifera oxygen isotope data (124) represented by a local regression smoother and associated 95% confidence intervals. Major tectonic and meteorite events are also indicated. (B) Relative extinction rate (extinction/speciation) (lines are medians, and light boxes around medians are interquartile ranges from 500 estimates from the tree posterior).
Similar articles
-
Mass extinction of birds at the Cretaceous-Paleogene (K-Pg) boundary.
Longrich NR, Tokaryk T, Field DJ. Longrich NR, et al. Proc Natl Acad Sci U S A. 2011 Sep 13;108(37):15253-7. doi: 10.1073/pnas.1110395108. Proc Natl Acad Sci U S A. 2011. PMID: 21914849 Free PMC article.
-
Bird evolution in the Eocene: climate change in Europe and a Danish fossil fauna.
Lindow BE, Dyke GJ. Lindow BE, et al. Biol Rev Camb Philos Soc. 2006 Nov;81(4):483-99. doi: 10.1017/S146479310600707X. Epub 2006 Aug 8. Biol Rev Camb Philos Soc. 2006. PMID: 16893476 Review.
-
Field DJ, Bercovici A, Berv JS, Dunn R, Fastovsky DE, Lyson TR, Vajda V, Gauthier JA. Field DJ, et al. Curr Biol. 2018 Jun 4;28(11):1825-1831.e2. doi: 10.1016/j.cub.2018.04.062. Epub 2018 May 24. Curr Biol. 2018. PMID: 29804807
-
The Paleogene fossil record of birds in Europe.
Mayr G. Mayr G. Biol Rev Camb Philos Soc. 2005 Nov;80(4):515-42. doi: 10.1017/S1464793105006779. Biol Rev Camb Philos Soc. 2005. PMID: 16221327 Review.
-
Pereira SL, Baker AJ. Pereira SL, et al. Mol Biol Evol. 2006 Sep;23(9):1731-40. doi: 10.1093/molbev/msl038. Epub 2006 Jun 14. Mol Biol Evol. 2006. PMID: 16774978
Cited by
-
Jiang C, Kang H, Zhou Y, Zhu W, Zhao X, Mohamed N, Li B. Jiang C, et al. Life (Basel). 2024 Jul 15;14(7):881. doi: 10.3390/life14070881. Life (Basel). 2024. PMID: 39063634 Free PMC article.
-
Signore AV, Tift MS, Hoffmann FG, Schmitt TL, Moriyama H, Storz JF. Signore AV, et al. Proc Natl Acad Sci U S A. 2021 Mar 30;118(13):e2023936118. doi: 10.1073/pnas.2023936118. Proc Natl Acad Sci U S A. 2021. PMID: 33753505 Free PMC article.
-
MEGA11: Molecular Evolutionary Genetics Analysis Version 11.
Tamura K, Stecher G, Kumar S. Tamura K, et al. Mol Biol Evol. 2021 Jun 25;38(7):3022-3027. doi: 10.1093/molbev/msab120. Mol Biol Evol. 2021. PMID: 33892491 Free PMC article.
-
Spaulding F, McLaughlin JF, Cheek RG, McCracken KG, Glenn TC, Winker K. Spaulding F, et al. Mol Phylogenet Evol. 2023 May;182:107733. doi: 10.1016/j.ympev.2023.107733. Epub 2023 Feb 16. Mol Phylogenet Evol. 2023. PMID: 36801373 Free PMC article.
-
Genome Sequence of Peacock Reveals the Peculiar Case of a Glittering Bird.
Jaiswal SK, Gupta A, Saxena R, Prasoodanan VPK, Sharma AK, Mittal P, Roy A, Shafer ABA, Vijay N, Sharma VK. Jaiswal SK, et al. Front Genet. 2018 Sep 19;9:392. doi: 10.3389/fgene.2018.00392. eCollection 2018. Front Genet. 2018. PMID: 30283495 Free PMC article.
References
-
- J. Cracraft, in The Howard & Moore Complete Checklist of the Birds of the World, Volume 1, E. C. Dickinson, L. Christidis, Eds. (Aves Press, Eastbourne, UK, ed. 4, 2013), pp. 21–43.
-
- J. Cracraft, in The Howard & Moore Complete Checklist of the Birds of the World, Volume 2, E. C. Dickinson, L. Christidis, Eds. (Aves Press, Eastbourne, UK, ed. 4, 2014), pp. 17–45.
-
- Jarvis E. D., Mirarab S., Aberer A. J., Li B., Houde F., Li C., Ho S. Y., Faircloth B. C., Nabholz B., Howard J. T., Suh A., Weber C. C., da Fonseca R. R., Li J., Zhang F., Li H., Zhou L., Narula N., Liu L., Ganapathy G., Boussau B., Bayzid M. S., Zavidovych V., Subramanian S., Gabaldón T., Capella-Gutiérrez S., Huerta-Cepas J., Rekepalli B., Munch K., Schierup M., Lindow B., Warren W. C., Ray D., Green R. E., Bruford M. W., Zhan X., Dixon A., Li S., Li N., Huang Y., Derryberry E. P., Bertelsen M. F., Sheldon F. H., Brumfield R. T., Mello C. V., Lovell P. V., Wirthlin M., Schneider M. P., Prosdocimi F., Samaniego J. A., Vargas Velazquez A. M., Alfaro-Núñez A., Campos P. F., Petersen B., Sicheritz-Ponten T., Pas A., Bailey T., Scofield P., Bunce M., Lambert D. M., Zhou Q., Perelman P., Driskell A. C., Shapiro B., Xiong Z., Zeng Y., Liu S., Li Z., Liu B., Wu K., Xiao J., Yinqi X., Zheng Q., Zhang Y., Yang H., Wang J., Smeds L., Rheindt F. E., Braun M., Fjeldsa J., Orlando L., Barker F. K., Jønsson K. A., Johnson W., Koepfli K. P., O’Brien S., Haussler D., Ryder O. A., Rahbek C., Willerslev E., Graves G. R., Glenn T. C., McCormack J., Burt D., Ellegren H., Alström P., Edwards S. V., Stamatakis A., Mindell D. P., Cracraft J., Braun E. L., Warnow T., Jun W., Gilbert M. T., Zhang G., Whole-genome analyses resolve early branches in the tree of life of modern birds. Science 346, 1320–1331 (2014). - PMC - PubMed
-
- Prum R. O., Berv J. S., Dornburg A., Field D. J., Townsend J. P., Lemmon E. M., Lemmon A. R., A comprehensive phylogeny of birds (Aves) using targeted next-generation DNA sequencing. Nature 526, 569–573 (2015). - PubMed
LinkOut - more resources
Full Text Sources
Other Literature Sources