Small genome of the fungus Escovopsis weberi, a specialized disease agent of ant agriculture - PubMed
- ️Fri Jan 01 2016
. 2016 Mar 29;113(13):3567-72.
doi: 10.1073/pnas.1518501113. Epub 2016 Mar 14.
Jason E Stajich 2 , Christian P Kubicek 3 , Clotilde Teiling 4 , Komal Chenthamara 3 , Lea Atanasova 3 , Irina S Druzhinina 3 , Natasha Levenkova 4 , Stephanie S L Birnbaum 1 , Seth M Barribeau 5 , Brooke A Bozick 1 , Garret Suen 6 , Cameron R Currie 6 , Nicole M Gerardo 7
Affiliations
- PMID: 26976598
- PMCID: PMC4822581
- DOI: 10.1073/pnas.1518501113
Small genome of the fungus Escovopsis weberi, a specialized disease agent of ant agriculture
Tom J B de Man et al. Proc Natl Acad Sci U S A. 2016.
Abstract
Many microorganisms with specialized lifestyles have reduced genomes. This is best understood in beneficial bacterial symbioses, where partner fidelity facilitates loss of genes necessary for living independently. Specialized microbial pathogens may also exhibit gene loss relative to generalists. Here, we demonstrate that Escovopsis weberi, a fungal parasite of the crops of fungus-growing ants, has a reduced genome in terms of both size and gene content relative to closely related but less specialized fungi. Although primary metabolism genes have been retained, the E. weberi genome is depleted in carbohydrate active enzymes, which is consistent with reliance on a host with these functions. E. weberi has also lost genes considered necessary for sexual reproduction. Contrasting these losses, the genome encodes unique secondary metabolite biosynthesis clusters, some of which include genes that exhibit up-regulated expression during host attack. Thus, the specialized nature of the interaction between Escovopsis and ant agriculture is reflected in the parasite's genome.
Keywords: Atta cephalotes; attine; genome reduction; mycoparasitism; repeat-induced point mutation.
Conflict of interest statement
The authors declare no conflict of interest.
Figures
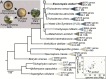
Escovopsis weberi, a specialized mycoparasite of the fungus-growing ant symbiosis, has a small genome compared with other Pezizomycotina fungi. (A) Both fungus-growing ants and the mycoparasite E. weberi use the ants’ cultivated fungi as their primary food source. The ability of the cultivated fungi to efficiently break down plant material gives both consumers access to the biomass of neotropical plants. (B) Size and protein-coding gene content of genomes of diverse fungi in the Pezizomycotina. Bayesian phylogeny estimated using partial amino acid alignments of three genes (Rpb1, Rpb2, ef1-α). All posterior probabilities are greater than 0.95. Phylogeny is rooted with Sacchormyces cervesiae (not shown). (C) Relationship between genome size and gene content. A list of genomes included in this panel is in SI Appendix, Table S1.
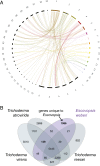
Similarities between Escovopsis and Trichoderma. (A) Mesosynteny between E. weberi and T. virens. Scaffolds of E. weberi are multicolored. T. virens scaffolds are black. Only scaffolds containing syntenic regions are shown. (B) Gene content overlap between E. weberi and three Trichoderma species. Like Escovopsis spp., Trichoderma spp. are mycoparasites (fungi that attack and consume other fungi), although they are less specialized and are also able to obtain nutrients from dead organic matter. Orthologs were assigned using all-against-all BLASTP for amino acids and inparanoid/multiparanoid (sequence overlap coverage ≥50%).
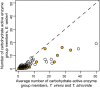
The E. weberi genome encodes a reduced number of carbohydrate active enzymes. Carbohydrate active enzymes are divided into families. Each point represents the relation between the number of members of a given CAZmye family for E. weberi plotted against the average number of family members for the less specialized mycoparasites T. virens and T. atroviride. Members of some of these families, indicated in orange, are known to be highly expressed in E. weberi’s host fungus (2, 3). Additional details are in SI Appendix, Table S10.
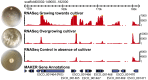
Up-regulation of gene expression within a secondary metabolite cluster during interaction with cultivated host fungi. Gbrowse genome browser view of 1 of 16 secondary metabolite clusters in the E. weberi genome. Below the scaffold are three tracks illustrating RNAseq-based gene expression when E. weberi is growing toward its host (Top), when it has overgrown its host (Middle), and in the absence of its host (Bottom); MAKER2 gene model predictions are illustrated below. Photographs next to each RNAseq track illustrate the growth of E. weberi under each condition. Each Petri dish was inoculated with the cultivated fungus near the top (when present) and E. weberi near the center 1 wk later; photographs were taken 3–4 d after E. weberi inoculation. Note that E. weberi grows much more rapidly in the presence than in the absence of its host. See SI Appendix, Fig. S1 for additional images.
Similar articles
-
Berasategui A, Salem H, Moller AG, Christopher Y, Vidaurre Montoya Q, Conn C, Read TD, Rodrigues A, Ziemert N, Gerardo N. Berasategui A, et al. mSystems. 2024 Jul 23;9(7):e0057624. doi: 10.1128/msystems.00576-24. Epub 2024 Jun 21. mSystems. 2024. PMID: 38904377 Free PMC article.
-
Symbiont-Mediated Host-Parasite Dynamics in a Fungus-Gardening Ant.
Kellner K, Kardish MR, Seal JN, Linksvayer TA, Mueller UG. Kellner K, et al. Microb Ecol. 2018 Aug;76(2):530-543. doi: 10.1007/s00248-017-1124-6. Epub 2017 Dec 28. Microb Ecol. 2018. PMID: 29285550
-
Chemical warfare between fungus-growing ants and their pathogens.
Batey SFD, Greco C, Hutchings MI, Wilkinson B. Batey SFD, et al. Curr Opin Chem Biol. 2020 Dec;59:172-181. doi: 10.1016/j.cbpa.2020.08.001. Epub 2020 Sep 17. Curr Opin Chem Biol. 2020. PMID: 32949983 Free PMC article. Review.
-
Symbiont recruitment versus ant-symbiont co-evolution in the attine ant-microbe symbiosis.
Mueller UG. Mueller UG. Curr Opin Microbiol. 2012 Jun;15(3):269-77. doi: 10.1016/j.mib.2012.03.001. Epub 2012 Mar 23. Curr Opin Microbiol. 2012. PMID: 22445196 Review.
Cited by
-
Genome analysis of the ubiquitous boxwood pathogen Pseudonectria foliicola.
Rivera Y, Salgado-Salazar C, Veltri D, Malapi-Wight M, Crouch JA. Rivera Y, et al. PeerJ. 2018 Aug 24;6:e5401. doi: 10.7717/peerj.5401. eCollection 2018. PeerJ. 2018. PMID: 30155349 Free PMC article.
-
Xu R, Liu X, Peng B, Liu P, Li Z, Dai Y, Xiao S. Xu R, et al. Pathogens. 2020 Mar 20;9(3):232. doi: 10.3390/pathogens9030232. Pathogens. 2020. PMID: 32245129 Free PMC article.
-
Wang Z, Kim W, Wang YW, Yakubovich E, Dong C, Trail F, Townsend JP, Yarden O. Wang Z, et al. Front Fungal Biol. 2023 Jun 30;4:1214537. doi: 10.3389/ffunb.2023.1214537. eCollection 2023. Front Fungal Biol. 2023. PMID: 37746130 Free PMC article. Review.
-
Conlon BH, Gostinčar C, Fricke J, Kreuzenbeck NB, Daniel JM, Schlosser MSL, Peereboom N, Aanen DK, de Beer ZW, Beemelmanns C, Gunde-Cimerman N, Poulsen M. Conlon BH, et al. iScience. 2021 Jun 1;24(6):102680. doi: 10.1016/j.isci.2021.102680. eCollection 2021 Jun 25. iScience. 2021. PMID: 34189441 Free PMC article.
-
Comparative genomics reveals a core gene toolbox for lifestyle transitions in Hypocreales fungi.
Wu B, Cox MP. Wu B, et al. Environ Microbiol. 2021 Jun;23(6):3251-3264. doi: 10.1111/1462-2920.15554. Epub 2021 May 11. Environ Microbiol. 2021. PMID: 33939870 Free PMC article.
References
-
- Weber NA. Fungus-growing ants. Science. 1966;153(3736):587–604. - PubMed
Publication types
MeSH terms
LinkOut - more resources
Full Text Sources
Other Literature Sources