Blood flow and mass transfer regulation of coagulation - PubMed
Review
Blood flow and mass transfer regulation of coagulation
Kuldeepsinh Rana et al. Blood Rev. 2016 Sep.
Abstract
Blood flow regulates coagulation and fibrin formation by controlling the transport, or mass transfer, of zymogens, co-factors, enzymes, and inhibitors to, from, and within a growing thrombus. The rate of mass transfer of these solutes relative to their consumption or production by coagulation reactions determines, in part, the rate of thrombin generation, fibrin deposition, and thrombi growth. Experimental studies on the influence of blood flow on specific coagulation reactions are reviewed here, along with a theoretical framework that predicts how flow influences surface-bound coagulation binding and enzymatic reactions. These flow-mediated transport mechanisms are also used to interpret the role of binding site densities and injury size on initiating coagulation and fibrin deposition. The importance of transport of coagulation proteins within the interstitial spaces of thrombi is shown to influence thrombi architecture, growth, and arrest.
Keywords: Biotransport; Coagulation; Hemorheology.
Copyright © 2016 Elsevier Ltd. All rights reserved.
Conflict of interest statement
None.
Figures
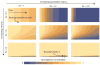
Flow moves from left to right. The substrate enters the domain on the left at a normalized concentration of unity (yellow) and is consumed by a binding reaction on the bottom wall leading to near-wall concentration of zero (blue). The binding reaction follows Langmuir kinetics (Eq. 1) . The velocity increases going down each column by one order-of-magnitude, and consequently increases the Peclet number (Pe, Eq. 6) by the same amount. Note the formation of a thin boundary layer of thickness δ near the bottom wall at Pe = 10, where convective flux is ten-fold higher than diffusive flux. The binding rate, kon, increases across each row by one order-of-magnitude, resulting in a higher Dahmkohler number (Da, Eq. 11). For Da greater than unity (middle and right columns), the substrate is completely consumed at the wall leading to a transport-limited regime.
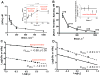
Purified solutions of zymogens (FX and prothrombin) are perfused through tubular bioreactors that consists of a capillary tube coated with lipid bilayers (PC:PS, 75:25) containing enzyme complexes (TF:FVIIa, FVa:FXa). The effluent is collected and the concentration of enzyme (FXa, thrombin) is measured as function of wall shear rate. A. Human FXa generation on 2.2 fmol/cm2 TF:FVIIa crosses over from a transport-limited regime to a reaction-limited regime at a wall shear rate of 500 s−1 owing to a reduction in the boundary layer thickness. This transition is characterize by an in increase in FXa flux with shear rate up to 500 s−1 (inset). B. Bovine thrombin generation on 1.7 fmol/cm2 FVa:FXa is reaction-limited over the entire range of shear rates. Reduction in thrombin concentration with increasing shear rate is due solely to dilution by flow. Note that the thrombin flux is constant over entire range of shear rates (inset). C. Double log plot of FXa and thrombin concentrations exiting the reactor as a function of flow velocity for isolated experiments with FX and thrombin. The slope of −1.2 for thrombin is indicative of a reaction-limited regime. The slope of −0.68 for FXa is indicative of a transport-limited regime (Eq. 5). D. Double log plot for simultaneous generation of FXa and thrombin in presence of both TF:FVIIa and FVa:FXa. The slope of −0.8 shows that the competition of FX for binding sites with prothrombin causes a transition in FXa production to a regime where transport rates and reaction rates are on the same order-of-magnitude.
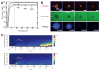
Experiments and simulations show that TF surface concentration or injury size must exceed a threshold value to yield a burst in thrombin generation characteristic of the propagation phase of coagulation. A. A computational models of thrombus formation under flow predict a threshold response in thrombin generation to surface TF concentrations that is a function of wall shear rate (100, 500, 1500 s−1). Note that above ~15 fmol/cm2 of TF that thrombin generation saturates suggesting reaction-limited behavior. B. Under static conditions, a threshold TF spots size is necessary to initiate coagulation from plasma. Below the threshold size coagulation products diffuse away from the spot before they can initiate robust thrombin generation. C. A computational model of plasma flowing over TF spots separated by TM coated walls. At low TM surface concentrations (top panel), thrombin generation is additive between adjacent TF sites because there is not enough APC to quench coagulation products between site. At high TM concentrations (bottom panel), the interaction between sites can be repressed.
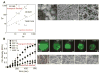
The mass transfer limitations on fibrin deposition are more stringent than thrombin owing to the multistep polymerization process. A. A state diagram (left) for fibrin morphology as a function of Pe (Eq. 6) and Da (Eq. 12) demonstrates regimes of transport-limited and reaction-limited fibrin deposition. The different morphologies are shown in electron micrographs (right) thin films, two-dimensional mats of fibers, and three dimensional gels. Scale bar = 1 μm. B. Fibrin deposition on TF coated silica beads during plasma perfusions at different shear rates (left). There is a transition from a transport-limited regime that supports three dimensional fibrin gel formation and a reaction-limited regime where fibrin fibers are limited to the interstitial spaces between the beads. Panels on the right show fluorescent images and electron micrographs of fibrin deposition at different shear rates.
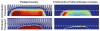
The access of zymogens to their enzymes in a growing thrombus can limit growth. Here, computational simulations of thrombus formation on a TF surface for cases of unhindered and hindered transport. In the left-hand column, colors indicate the platelet density from plasma concentration (blue) to dense packing (red). In the right-hand column, colors indicate the concentration of prothrombin bound to prothrombinase from low (blue) to high (red). Coagulation factor transport that is hindered due to reduced interstitial space between platelets that limits the ability of prothrombin to penetrate into the thrombus and reach platelet-bound prothrombinase. Note that hindered transport leads to a denser, smaller thrombi compared to unhindered transport.
Similar articles
-
Platelet Control of Fibrin Distribution and Microelasticity in Thrombus Formation Under Flow.
Swieringa F, Baaten CC, Verdoold R, Mastenbroek TG, Rijnveld N, van der Laan KO, Breel EJ, Collins PW, Lancé MD, Henskens YM, Cosemans JM, Heemskerk JW, van der Meijden PE. Swieringa F, et al. Arterioscler Thromb Vasc Biol. 2016 Apr;36(4):692-9. doi: 10.1161/ATVBAHA.115.306537. Epub 2016 Feb 4. Arterioscler Thromb Vasc Biol. 2016. PMID: 26848157
-
Swieringa F, Kuijpers MJ, Lamers MM, van der Meijden PE, Heemskerk JW. Swieringa F, et al. Haematologica. 2015 Jun;100(6):748-56. doi: 10.3324/haematol.2014.116863. Epub 2015 Mar 13. Haematologica. 2015. PMID: 25769543 Free PMC article.
-
Fogelson AL, Tania N. Fogelson AL, et al. Pathophysiol Haemost Thromb. 2005;34(2-3):91-108. doi: 10.1159/000089930. Pathophysiol Haemost Thromb. 2005. PMID: 16432311
-
Rheology of blood coagulation.
Kaibara M. Kaibara M. Biorheology. 1996 Mar-Apr;33(2):101-17. doi: 10.1016/0006-355X(96)00010-8. Biorheology. 1996. PMID: 8679959 Review.
-
Physiology of blood coagulation.
Bloom AL. Bloom AL. Haemostasis. 1990;20 Suppl 1:14-29. doi: 10.1159/000216159. Haemostasis. 1990. PMID: 2083865 Review.
Cited by
-
The Art and Science of Building a Computational Model to Understand Hemostasis.
Leiderman K, Sindi SS, Monroe DM, Fogelson AL, Neeves KB. Leiderman K, et al. Semin Thromb Hemost. 2021 Mar;47(2):129-138. doi: 10.1055/s-0041-1722861. Epub 2021 Feb 26. Semin Thromb Hemost. 2021. PMID: 33657623 Free PMC article. Review.
-
A MATHEMATICAL MODEL OF PLATELET AGGREGATION IN AN EXTRAVASCULAR INJURY UNDER FLOW.
Link KG, Sorrells MG, Danes NA, Neeves KB, Leiderman K, Fogelson AL. Link KG, et al. Multiscale Model Simul. 2020;18(4):1489-1524. doi: 10.1137/20m1317785. Epub 2020 Nov 18. Multiscale Model Simul. 2020. PMID: 33867873 Free PMC article.
-
Tomaiuolo M, Brass LF, Stalker TJ. Tomaiuolo M, et al. Interv Cardiol Clin. 2017 Jan;6(1):1-12. doi: 10.1016/j.iccl.2016.08.001. Interv Cardiol Clin. 2017. PMID: 27886814 Free PMC article. Review.
-
A microfluidic method to investigate platelet mechanotransduction under extensional strain.
Zainal Abidin NA, Timofeeva M, Szydzik C, Akbaridoust F, Lav C, Marusic I, Mitchell A, Hamilton JR, Ooi ASH, Nesbitt WS. Zainal Abidin NA, et al. Res Pract Thromb Haemost. 2023 Jan 10;7(1):100037. doi: 10.1016/j.rpth.2023.100037. eCollection 2023 Jan. Res Pract Thromb Haemost. 2023. PMID: 36846647 Free PMC article.
-
The biophysics and mechanics of blood from a materials perspective.
Qiu Y, Myers DR, Lam WA. Qiu Y, et al. Nat Rev Mater. 2019 May;4(5):294-311. doi: 10.1038/s41578-019-0099-y. Epub 2019 Mar 28. Nat Rev Mater. 2019. PMID: 32435512 Free PMC article.
References
-
- Davie EW, Fujikawa K, Kisiel W. The coagulation cascade: initiation, maintenance, and regulation. Biochemistry. 1991;30(43):10363–70. - PubMed
-
- Monroe D, Hoffman M. What Does It Take to Make the Perfect Clot? Arterioscl Throm Vas. 2006;26(1):41. - PubMed
-
- Owen CA., Jr . In: A History of Blood Coagulation. Nichols WL, Walter Bowie EJ, editors. Rochester, MN: Mayo Foundation for Medical Education and Research; 2001.
-
- Gaetano G, de Cerletti C. Platelet adhesion and aggregation and fibrin formation in flowing blood: a historical contribution by Giulio Bizzozero. Platelets. 2002;13(2):85–9. - PubMed
Publication types
MeSH terms
LinkOut - more resources
Full Text Sources
Other Literature Sources
Molecular Biology Databases