Non integrative strategy decreases chromosome instability and improves endogenous pluripotency genes reactivation in porcine induced pluripotent-like stem cells - PubMed
- ️Fri Jan 01 2016
Harmonie Barasc 2 , Kamila Canale-Tabet 1 , Florence Plisson-Petit 1 , Chantal Delcros 1 , Olivier Feraud 3 , Noufissa Oudrhiri 3 4 , Eva Hadadi 3 , Franck Griscelli 3 5 6 , Annelise Bennaceur-Griscelli 3 4 7 , Ali Turhan 3 7 , Marielle Afanassieff 8 , Stéphane Ferchaud 9 , Alain Pinton 2 , Martine Yerle-Bouissou 1 , Hervé Acloque 1
Affiliations
- PMID: 27245508
- PMCID: PMC4887982
- DOI: 10.1038/srep27059
Non integrative strategy decreases chromosome instability and improves endogenous pluripotency genes reactivation in porcine induced pluripotent-like stem cells
Annabelle Congras et al. Sci Rep. 2016.
Erratum in
-
Congras A, Barasc H, Canale-Tabet K, Plisson-Petit F, Delcros C, Feraud O, Oudrhiri N, Hadadi E, Griscelli F, Bennaceur-Griscelli A, Turhan A, Afanassieff M, Ferchaud S, Pinton A, Yerle-Bouissou M, Acloque H. Congras A, et al. Sci Rep. 2018 Jan 8;8:46931. doi: 10.1038/srep46931. Sci Rep. 2018. PMID: 29308785 Free PMC article.
Abstract
The pig is an emerging animal model, complementary to rodents for basic research and for biomedical and agronomical purposes. However despite the progress made on mouse and rat models to produce genuine pluripotent cells, it remains impossible to produce porcine pluripotent cell lines with germline transmission. Reprogramming of pig somatic cells using conventional integrative strategies remains also unsatisfactory. In the present study, we compared the outcome of both integrative and non-integrative reprogramming strategies on pluripotency and chromosome stability during pig somatic cell reprogramming. The porcine cell lines produced with integrative strategies express several pluripotency genes but they do not silence the integrated exogenes and present a high genomic instability upon passaging. In contrast, pig induced pluripotent-like stem cells produced with non-integrative reprogramming system (NI-iPSLCs) exhibit a normal karyotype after more than 12 months in culture and reactivate endogenous pluripotency markers. Despite the persistent expression of exogenous OCT4 and MYC, these cells can differentiate into derivatives expressing markers of the three embryonic germ layers and we propose that these NI-iPSLCs can be used as a model to bring new insights into the molecular factors controlling and maintaining pluripotency in the pig and other non-rodent mammalians.
Figures
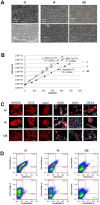
(A) Phase contrast image of I3, I4 and I20 lines harboring an iPS-like morphology on feeder cells. (B) Proliferation curve of I3, I4 and I20 lines on feeder cells. (C) Immunoflurorescence analysis revealing the nuclear expression of NANOG, OCT4 and SOX2 (Alexa Fluor (AF) 594) and the extra-nuclear expression of LIN28, CDH1 and SSEA4 (AF594 and DAPI nuclei counterstaining), scale bar: 25 μm. (D) Flow cytometry immunofluorescence analysis indicates the expression of pluripotency-related surface antigen SSEA4 in a subpopulation of cells (around 55% in I3, 35% in I4, 18% in I20) while SSEA3 and TRA160 are less represented.
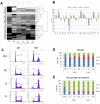
(A) I3 and I4 lines share a close expression profile of pluripotency-related genes depending on the culture media while the expression profile of I20 and non-reprogrammed cell lines are more distant. Black and white gradations highlight downregulated and upregulated genes, respectively. (B) Variation in expression level in a subset of pluripotency-related genes in I3 and I4 lines upon transition to the LIF+2i medium. Results are normalized on the expression in the I3 line cultured in bFGF-containing medium (log scale, t-test *p < 0.05 **p < 0.01 ***p < 0.001). (C) Cytograms representing the distribution of cells in each phase of the cellular cycle in non-reprogrammed and reprogrammed cell lines with or without double strand break induction (M1 = G1 phase; M2 = S phase; M3 = G2/M phase; DH = Doxorubicin hydrochloride). (D) Shortened G1 phase and extended S phase in reprogrammed lines cultured either in piPS or LIF+2i culture media compared to non-reprogrammed PEFs. (E) Absence of the G1/S cell cycle checkpoint in I lines assessed by the non-retention of cells in G1 phase after DSB induction by DH treatment compared to the PEF cell distribution.
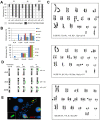
(A) Increase of abnormal karyotypes with passaging in I3, I4 and I20 lines. Light grey represents the percentage of normal karyotypes and dark grey the percentage of abnormal karyotypes. (B) Evolution of the main rearrangements observed in I3 and I20 lines. (C) Examples of the most represented abnormal karyotypes in I3, I4 and I20 lines at late passages. Arrows indicate the rearrangements. (D) Identification of common genomic duplication on chromosomes 3, 4 and 10 in both I3 and I4 lines by CGH analysis (green lines = duplications; red lines = deletions). (E) FISH analysis using painting probes SSC8 (green) and SSC4 (red) revealed the translocation of a duplicated fragment of chromosome 4 at the extremity of chromosome 8 with was not labeled by SSC8 probe (yellow arrow).
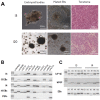
(A) Embryoid bodies formed by I3 and I20 cell lines poorly differentiate after 5 days of floating culture followed by 10 days in adherent conditions. Teratoma assay leads to the formation of undifferentiated adenocarcinomas. (B) Continuous expression of pluripotency genes in the embryoid bodies. (C) Continuous expression of exogenous genes in both bFGF and LIF+2i containing media and upon embryoid bodies formation.
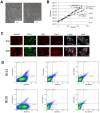
(A) iPS-like morphology of NI13 and NI20 lines on feeder cells, scale bar 30 μm. (B) NI cell lines grew slightly slower than I20 line. (C) Immunofluorescence analysis reveals the nuclear expression of NANOG, OCT4, SOX2 and SALL4 (AF594) and the extra-nuclear expression of LIN28 and CDH1 (AF594 and DAPI nuclei counterstaining) in both NI13 and NI20 lines, scale bar 25 μm. (D) Flow cytometry immunofluorescence analysis revealing the strong SSEA1 expression in NI13 and NI20 lines (respectively 50.5% and 60.5%) while SSEA4, TRA160 and SSEA3 are not expressed (less than 3% of the cell population).
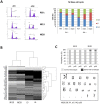
(A) Distribution of cells in the three phases of the cell cycle (M1 = G1 phase; M2 = S phase; M3 = G2/M phase) in PEFs and NI indicating a slight shortening of G1 phase duration in NI lines compared to PEFs, the presence of the G1/S checkpoint in both PEF and NI lines, and the extension of the S phase in NI lines after DSB induction. (B) NI lines harbor a stronger expression of the pluripotency-related genes than I lines. Black and white gradations highlight downregulated and upregulated genes, respectively. (C) Chromosomal stability of NI lines is not affected by time in culture. Light grey represents the percentage of normal karyotypes and dark grey the percentage of abnormal karyotypes.
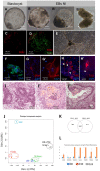
(A–D) NI-iPSLCs are able to form embryoid bodies with different morphologies (B,B”) some of them resembling porcine blastocysts (A,B,B’) as they harbor a dense cell mass (like in B,B’) expressing SOX2 (C) and a cellular envelop expressing CDX2 (D). (E–H) In adherent culture conditions, embryoid bodies derived from NI lines differentiate in several cellular types with various morphologies (E). Immunostaining for 3-germ layer specific markers highlight the presence of endodermal cells ((F) clumps of alpha-fetoprotein (AFP) positive cells in green), of mesodermal cells ((G,G’) scattered smooth muscle actin (SMA) positive cells in red) or ectodermal cells ((H,H’) small group of cells with neurons morphology and positive for beta-III tubulin (TUJ1) expression in red, white arrowheads highlight neuron-like projections). (I) Histological analysis of teratoma formed by NI lines shows the differentiation into the three germ layers with ectoderm-derived neural crests (I), mesoderm-derived chondrocytes with osteoid substance (I’) and endoderm-derived epithelium (I”). (J) Principal Component Analysis of microarray transcriptomic data showing that the repartition of the different expression profiles is explained by the reprogramming technique ((I) lines, I20, NI lines) and the level of reprogrammation (reprogrammed, embryoid bodies, non-reprogrammed). (K) A large number of differentially expressed probes between NI lines and their respective EB are common for NI13 and NI20 lines. (L) Dynamic variation in the expression level of pluripotency-related genes at D0 (blue bars), D10 (orange bars) and D24 (grey bars) of differentiation.
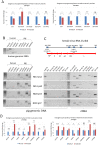
(A) Real-Time PCR were performed on cDNAs produced from NI13 and NI20 cell lines at early (p7, blue bars) and late (respectively p29 and p33, red bars) passages. Expression of exogenous factors hsOCT4 (SeV OCT4), hsSOX2 (SeV SOX2), hs KLF4 (SeV KLF4), hsMYC (SeV MYC) and Sendai virus RNA (SeV) was quantified between early and late passages in culture. Data are means and SD of two independent experiments (t-test, *P < 0.05; **P < 0.01; ***P < 0.001, NS: not significant). (B) PCR analysis was performed on human and pig genomic DNA with primers recognizing specifically the coding sequence of human or pig pluripotency genes. 40 amplification cycles were performed on genomic DNA from human cells or NI13 and NI20 cells at early and late passages. Human sequences were never amplified in the genomic DNA from NI-iPSLCs. (C) Representation of the Sendai virus genome and map of the primers used in the PCR analysis. Primers for detecting reprogramming genes are located on one side in the Sendai genome and on the other side in the coding sequence of reprogramming factors, depending on their insertion sites. Three pairs of primers amplifying Sendai virus genes are also mapped. PCRs were performed on genomic DNA from NI13 and NI20 cell lines at early and late passages for 40 amplification cycles. Insertion of exogenous factors hsOCT4 (SeV OCT4), hsSOX2 (SeV SOX2), hs KLF4 (SeV KLF4), hsMYC (SeV MYC) and Sendai virus genes (SeV, SeV L and SeV HN) was never detected. Oppositely, RT-PCRs using RNAs extracted from NI13 and NI20 cell lines at early and late passages clearly show that the whole Sendai virus RNA is expressed and is maintained in the cytoplasm of pig NI-iPSLCs. hsOCT4 and hsMYC expression is maintained among passages, while hsSOX2 and hsKLF4 are not detectable with this assay. (D) Real-Time PCR were performed on cDNAs produced from NI13 and NI20 cell lines at early (p7, blue bars) and late (respectively p29 and p33, red bars) passages to quantify relative expression of endogenous pluripotency markers ssOCT4, ssSOX2, ssKLF4, ssMYC, ssSALL4, ssNANOG, ssESRRB and ssLIN28. Data are means and SD of two independent experiments (t-test, *P < 0.05; **P < 0.01; ***P < 0.001, NS: not significant).
Similar articles
-
Chen F, Zhang G, Yu L, Feng Y, Li X, Zhang Z, Wang Y, Sun D, Pradhan S. Chen F, et al. Stem Cell Res Ther. 2016 Jul 30;7(1):99. doi: 10.1186/s13287-016-0358-4. Stem Cell Res Ther. 2016. PMID: 27473118 Free PMC article.
-
Okada M, Yoneda Y. Okada M, et al. Biochim Biophys Acta. 2011 Feb;1810(2):226-35. doi: 10.1016/j.bbagen.2010.10.004. Epub 2010 Oct 20. Biochim Biophys Acta. 2011. PMID: 20965232
-
Derivation of induced pluripotent stem cells from orangutan skin fibroblasts.
Ramaswamy K, Yik WY, Wang XM, Oliphant EN, Lu W, Shibata D, Ryder OA, Hacia JG. Ramaswamy K, et al. BMC Res Notes. 2015 Oct 16;8:577. doi: 10.1186/s13104-015-1567-0. BMC Res Notes. 2015. PMID: 26475477 Free PMC article.
-
Brouwer M, Zhou H, Nadif Kasri N. Brouwer M, et al. Stem Cell Rev Rep. 2016 Feb;12(1):54-72. doi: 10.1007/s12015-015-9622-8. Stem Cell Rev Rep. 2016. PMID: 26424535 Free PMC article. Review.
-
An Overview of Direct Somatic Reprogramming: The Ins and Outs of iPSCs.
Menon S, Shailendra S, Renda A, Longaker M, Quarto N. Menon S, et al. Int J Mol Sci. 2016 Jan 21;17(1):141. doi: 10.3390/ijms17010141. Int J Mol Sci. 2016. PMID: 26805822 Free PMC article. Review.
Cited by
-
Derivation of induced pluripotent stem cells from ferret somatic cells.
Gao J, Petraki S, Sun X, Brooks LA, Lynch TJ, Hsieh CL, Elteriefi R, Lorenzana Z, Punj V, Engelhardt JF, Parekh KR, Ryan AL. Gao J, et al. Am J Physiol Lung Cell Mol Physiol. 2020 Apr 1;318(4):L671-L683. doi: 10.1152/ajplung.00456.2019. Epub 2020 Feb 19. Am J Physiol Lung Cell Mol Physiol. 2020. PMID: 32073882 Free PMC article.
-
Epigenetic Modifiers Facilitate Induction and Pluripotency of Porcine iPSCs.
Mao J, Zhang Q, Deng W, Wang H, Liu K, Fu H, Zhao Q, Wang X, Liu L. Mao J, et al. Stem Cell Reports. 2017 Jan 10;8(1):11-20. doi: 10.1016/j.stemcr.2016.11.013. Epub 2016 Dec 29. Stem Cell Reports. 2017. PMID: 28041878 Free PMC article.
-
The progress of induced pluripotent stem cells derived from pigs: a mini review of recent advances.
Neira JA, Conrad JV, Rusteika M, Chu LF. Neira JA, et al. Front Cell Dev Biol. 2024 Jun 24;12:1371240. doi: 10.3389/fcell.2024.1371240. eCollection 2024. Front Cell Dev Biol. 2024. PMID: 38979033 Free PMC article. Review.
-
Capturing Human Naïve Pluripotency in the Embryo and in the Dish.
Zimmerlin L, Park TS, Zambidis ET. Zimmerlin L, et al. Stem Cells Dev. 2017 Aug 15;26(16):1141-1161. doi: 10.1089/scd.2017.0055. Epub 2017 Jun 26. Stem Cells Dev. 2017. PMID: 28537488 Free PMC article. Review.
-
Cerebral organoids and their potential for studies of brain diseases in domestic animals.
Pain B, Baquerre C, Coulpier M. Pain B, et al. Vet Res. 2021 May 3;52(1):65. doi: 10.1186/s13567-021-00931-z. Vet Res. 2021. PMID: 33941270 Free PMC article. Review.
References
-
- Takahashi K. & Yamanaka S. Induction of pluripotent stem cells from mouse embryonic and adult fibroblast cultures by defined factors. Cell. 126, 663–676 (2006). - PubMed
-
- Takahashi K. et al.. Induction of pluripotent stem cells from adult human fibroblasts by defined factors. Cell 131, 861–872 (2007). - PubMed
-
- Ezashi T., Telugu B. P. & Roberts R. M. Induced pluripotent stem cells from pigs and other ungulate species: an alternative to embryonic stem cells? Reprod Domest Anim 47, 92–97 (2012). - PubMed
-
- Brevini T. A., Antonini S., Cillo F., Crestan M. & Gandolfi F. Porcine embryonic stem cells: Facts, challenges and hopes. Theriogenology 68, S206–213 (2007). - PubMed
-
- Blomberg L. A. & Telugu B. P. Twenty years of embryonic stem cell research in farm animals. Reprod Domest Anim 47, 80–85 (2012). - PubMed
Publication types
MeSH terms
Substances
LinkOut - more resources
Full Text Sources
Other Literature Sources