The Calcium-Sensing Receptor and Integrins in Cellular Differentiation and Migration - PubMed
- ️Fri Jan 01 2016
Review
The Calcium-Sensing Receptor and Integrins in Cellular Differentiation and Migration
Sujeenthar Tharmalingam et al. Front Physiol. 2016.
Abstract
The calcium-sensing receptor (CaSR) is a widely expressed homodimeric G-protein coupled receptor structurally related to the metabotropic glutamate receptors and GPRC6A. In addition to its well characterized role in maintaining calcium homeostasis and regulating parathyroid hormone release, evidence has accumulated linking the CaSR with cellular differentiation and migration, brain development, stem cell engraftment, wound healing, and tumor growth and metastasis. Elevated expression of the CaSR in aggressive metastatic tumors has been suggested as a potential novel prognostic marker for predicting metastasis, especially to bone tissue where extracellular calcium concentrations may be sufficiently high to activate the receptor. Recent evidence supports a model whereby CaSR-mediated activation of integrins promotes cellular migration. Integrins are single transmembrane spanning heterodimeric adhesion receptors that mediate cell migration by binding to extracellular matrix proteins. The CaSR has been shown to form signaling complexes with the integrins to facilitate both the movement and differentiation of cells, such as neurons during normal brain development and tumor cells under pathological circumstances. Thus, CaSR/integrin complexes may function as a universal cell migration or homing complex. Manipulation of this complex may be of potential interest for treating metastatic cancers, and for developmental disorders pertaining to aberrant neuronal migration.
Keywords: G-protein coupled receptor; GPRC6A; calcium; cerebellar granule cells; cerebellum; extracellular matrix; medulloblastoma; metastasis.
Figures
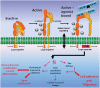
Integrin structure and bidirectional signaling. Integrins are membrane-spanning glycoprotein heterodimers that mediate cell adhesion and migration. The large extracellular domains contain sites for ligand and cation binding, while the small intracellular region senses changes in intracellular signaling pathways that convey information to the ECM via conformational modifications. Inactive integrins are present in a bent conformation (low affinity, left). Intracellular activation signals induce integrins to attain an upright position with a dual extended head-piece arrangement (middle). The upright conformation is characterized by an intermediate affinity state and a high affinity, ligand-bound state where the integrin α and β subunit tails are separated. Integrin inside-out signaling is achieved when intracellular signaling proteins push the β integrin leg away from the α integrin subunit, allowing for formation of an integrin high affinity activation state (right). Stabilization of integrin binding to ECM initiates outside-in signaling, where the integrins mediate downstream signaling that includes focal adhesion and actin stress fiber formations, activation of Rho GTPases, and cellular structural changes, etc., which ultimately leads to promotion of cell adhesion and migration.
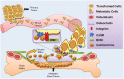
CaSR and integrins in cancer metastasis to bone. The diagram illustrates a proposed multi-step process whereby primary tumor cells enter the vasculature and colonize resorbing regions in bone. Tumor cells with elevated CaSR and integrin expression intravastates blood vessels and circulate in the bloodstream. Resorbing bone houses osteoclasts which release Ca2+; elevated extracellular Ca2+ is detected by the CaSR which activates integrin heterodimers to promote binding to ECM proteins such as collagen and laminin.
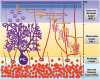
Cerebellar development and GCP migration (adapted from Tharmalingam, 2014). GCPs (blue spheres, top) proliferate in response to growth signals including sonic hedgehog (shh) released by Purkinje cells (PC, purple). Mitotic GCPs exit the cell cycle to become migratory post-mitotic bipolar GCPs (orange) that undergo tangential migration in the external granule-cell layer (EGL). The bipolar migratory GCPs sprout a third process and attach to Bergmann glia (BG) fibers (red) to undergo radial migration through the molecular layer (ML) and the Purkinje layer (PL), and into the internal granule-cell layer (IGL; mature granule neurons, dark orange). GCPs undergo radial migration by sensing extracellular matrix cues (laminin, vitronectin, etc.) and radially expanding transglial Ca2+ waves (pink circles) released by Bergmann glial processes.
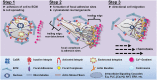
Proposed mechanism for CaSR-integrin mediated directional cell migration toward a CaSR-agonist gradient. Step 1: under resting conditions there is a homogenous distribution of integrins along the plasma membrane of the cell. Integrins present in the active confirmation are bound to the ECM proteins allowing the cell to spread evenly on the ECM substratum. In the absence of CaSR agonists, the integrins present in the CaSR-integrin complex adopt an inactive bent conformation. Step 2: exposure of the cell to a Ca2+ gradient results in CaSR-integrin mediated cell polarization. The CaSR-integrin complexes present proximal to the Ca2+ gradient uses the CaSR to sense the stimulus allowing for localized activation of intracellular signaling pathways. Activation of CaSR triggers PLC-IP3 mediated rise in intracellular Ca2+ causing integrin inside-out activation and elevated activated integrins at the site of CaSR stimulation. CaSR activation also promotes ERK2 and Akt-mediated trafficking and insertion of cytoplasmic endosomal integrins into the plasma membrane. Increased integrin-ECM interactions at the leading edge establishes cell polarity by allowing the formation of adhesion contacts and actin polymerization at the migrating front, while the tailing edge disassembles actin formation. Step 3: CaSR stimulation dependent redistribution of integrins and actin stress formation from the trailing edges to the migrating front is important for guided cell migration. The transfer of actin fibers to the leading edge of the cell, while limiting the level of integrins, actin and microtubules available in the retracting edge prompts directed cell migration toward the calcium gradient. The dynamic assembly and disassembly, together with actomyosin contractility moves the cell toward the Ca2+ gradient.
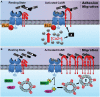
Potential mechanisms of CaSR-integrin actions in cancer cells and the developing CNS. (A) (adapted from Tharmalingam et al., 2011) depicts one possible scenario where the CaSR is associated with integrin heterodimers in a signaling complex in thyroid carcinoma cells. Left, the complex prior to CaSR stimulation; right, elevated extracellular calcium activate the CaSR causing its Venus flytrap domain to undergo a conformational change. This protein domain movement may induce integrins to flip it into an activated state thereby promoting binding to the ECM and enhanced cellular migration. The “X” denotes a hypothetical protein that may participate in facilitating the CaSR-mediated activation of integrins subsequent to increased [Ca 2+]i. (B) (adapted from Tharmalingam et al., 2016) depicts cross-talk between CaSR (blue), integrin heterodimers (red), and intracellular protein kinases in the developing cerebellum. Left, the CaSR/integrin complex prior to stimulation; right, elevated extracellular Ca2+ (and/or polyamines) activate the CaSR which then increases phosphorylation of ERK2 and Akt promoting β1 integrin cell surface expression. The accumulation of integrins at the leading edge of GCPs facilitates stable attachments between the GCPs and the ECM and enhances cellular migration.
Similar articles
-
Tharmalingam S, Wu C, Hampson DR. Tharmalingam S, et al. Dev Neurobiol. 2016 Apr;76(4):375-89. doi: 10.1002/dneu.22321. Epub 2015 Jul 16. Dev Neurobiol. 2016. PMID: 26138678
-
Calcium-sensing receptor modulates cell adhesion and migration via integrins.
Tharmalingam S, Daulat AM, Antflick JE, Ahmed SM, Nemeth EF, Angers S, Conigrave AD, Hampson DR. Tharmalingam S, et al. J Biol Chem. 2011 Nov 25;286(47):40922-33. doi: 10.1074/jbc.M111.265454. Epub 2011 Oct 3. J Biol Chem. 2011. PMID: 21969374 Free PMC article.
-
Diverse roles of extracellular calcium-sensing receptor in the central nervous system.
Bandyopadhyay S, Tfelt-Hansen J, Chattopadhyay N. Bandyopadhyay S, et al. J Neurosci Res. 2010 Aug 1;88(10):2073-82. doi: 10.1002/jnr.22391. J Neurosci Res. 2010. PMID: 20336672 Review.
-
Ray K. Ray K. Prog Mol Biol Transl Sci. 2015;132:127-50. doi: 10.1016/bs.pmbts.2015.02.006. Epub 2015 Apr 25. Prog Mol Biol Transl Sci. 2015. PMID: 26055057 Review.
-
Calcium-sensing receptor (CaSR) promotes development of bone metastasis in renal cell carcinoma.
Frees S, Breuksch I, Haber T, Bauer HK, Chavez-Munoz C, Raven P, Moskalev I, D Costa N, Tan Z, Daugaard M, Thüroff JW, Haferkamp A, Prawitt D, So A, Brenner W. Frees S, et al. Oncotarget. 2018 Mar 2;9(21):15766-15779. doi: 10.18632/oncotarget.24607. eCollection 2018 Mar 20. Oncotarget. 2018. PMID: 29644008 Free PMC article.
Cited by
-
Yuan H, Yang G, Li S, Li L, Wei T, Song G, Luan H, Meng J, Wang Q, Yu Y, Sun J. Yuan H, et al. Iran J Basic Med Sci. 2020 Oct;23(10):1353-1359. doi: 10.22038/ijbms.2020.47436.10916. Iran J Basic Med Sci. 2020. PMID: 33149870 Free PMC article.
-
Hypercalcemia in Cancer: Causes, Effects, and Treatment Strategies.
Bartkiewicz P, Kunachowicz D, Filipski M, Stebel A, Ligoda J, Rembiałkowska N. Bartkiewicz P, et al. Cells. 2024 Jun 18;13(12):1051. doi: 10.3390/cells13121051. Cells. 2024. PMID: 38920679 Free PMC article. Review.
-
Kornsuthisopon C, Nowwarote N, Chansaenroj A, Photichailert S, Rochanavibhata S, Klincumhom N, Petit S, Dingli F, Loew D, Fournier BPJ, Osathanon T. Kornsuthisopon C, et al. Sci Rep. 2024 Mar 21;14(1):6777. doi: 10.1038/s41598-024-56845-1. Sci Rep. 2024. PMID: 38514682 Free PMC article.
-
Yuan H, Xu J, Zhu Y, Li L, Wang Q, Yu Y, Zhou B, Liu Y, Xu X, Wang Z. Yuan H, et al. Mol Med Rep. 2020 Sep;22(3):2021-2031. doi: 10.3892/mmr.2020.11277. Epub 2020 Jun 26. Mol Med Rep. 2020. PMID: 32705187 Free PMC article.
-
Wang Y, Gao P, Wei C, Li H, Zhang L, Zhao Y, Wu B, Tian Y, Zhang W, Wu L, Wang R, Xu C. Wang Y, et al. Cell Death Dis. 2017 May 18;8(5):e2799. doi: 10.1038/cddis.2017.193. Cell Death Dis. 2017. PMID: 28518143 Free PMC article.
References
-
- Aguirre A., González A., Navarro M., Castaño Ó., Planell J. A., Engel E. (2012). Control of microenvironmental cues with a smart biomaterial composite promotes endothelial progenitor cell angiogenesis. Eur. Cell. Mater. 24, 90–106. discussion: 106. - PubMed
Publication types
LinkOut - more resources
Full Text Sources
Other Literature Sources