Multi-functionality Redefined with Colloidal Carotene Carbon Nanoparticles for Synchronized Chemical Imaging, Enriched Cellular Uptake and Therapy - PubMed
- ️Fri Jan 01 2016
Multi-functionality Redefined with Colloidal Carotene Carbon Nanoparticles for Synchronized Chemical Imaging, Enriched Cellular Uptake and Therapy
Santosh K Misra et al. Sci Rep. 2016.
Abstract
Typically, multiplexing high nanoparticle uptake, imaging, and therapy requires careful integration of three different functions of a multiscale molecular-particle assembly. Here, we present a simpler approach to multiplexing by utilizing one component of the system for multiple functions. Specifically, we successfully synthesized and characterized colloidal carotene carbon nanoparticle (C(3)-NP), in which a single functional molecule served a threefold purpose. First, the presence of carotene moieties promoted the passage of the particle through the cell membrane and into the cells. Second, the ligand acted as a potent detrimental moiety for cancer cells and, finally, the ligands produced optical contrast for robust microscopic detection in complex cellular environments. In comparative tests, C(3)-NP were found to provide effective intracellular delivery that enables both robust detection at cellular and tissue level and presents significant therapeutic potential without altering the mechanism of intracellular action of β-carotene. Surface coating of C(3) with phospholipid was used to generate C(3)-Lipocoat nanoparticles with further improved function and biocompatibility, paving the path to eventual in vivo studies.
Figures
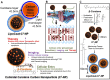
(A) Graphical representation of a multifunctional nanoparticle system in anhydrous state presenting carotene functionalities on the surface for synchronous imaging, therapy and cellular transport. (B) Schematic portraying CNPs containing carotenoids driven into the cellular phospholipid bilayer. (C) Assembly of C3-NP from molecular to nanoscale, showing the final particle of use.
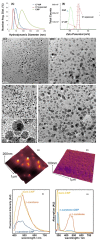
(A) Hydrodynamic diameter; (B) Zeta potential; anhydrous state size of (C,D) C3-NPs and (E,F) C3 Lipocoat nanoparticles by TEM; (G,H) height profile of C3-NPs by AFM. The spectroscopic evaluation of integral photonic properties of C3-NPs and compared to bare-CNP and β-carotene itself for (I) fluorescence properties and (J) UV-vis absorption efficiency. Properties were well compared with bare-CNP and β-carotene formulations to show the co-existence in C3 particles.
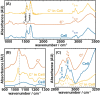
Panel A shows the baseline corrected IR spectrum of a typical cell, C3 and C3 in cells. Spectra are offset for clarity. Panels B and C show the details of the IR spectra of the same in two different spectral regions. Characteristic spectral features of C3 are observed in cells as shown in Panel B at 1045 cm−1. Panel C displays the increase in the peak intensity ratio between ν2922 and ν2957 cm−1.
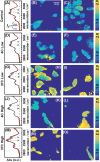
IR images of the cell and C3 incubated in cells for 5 cases as mentioned in the rows. (A,D,G,J,M) Show the average, baseline corrected IR spectra with the variance, while two separate images corresponding to each of the conditions are displayed in the rest. The intensity of each pixel is calculated as the ratio between 2922 and 2958 cm−1 band.
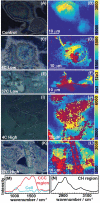
(A,C,E,I,K) Display the bright field images of breast cancer cells incubated with C3 at 5 different conditions. Raman images of the same regions are shown in (B,D,F,J,L) respectively. The C-H region intensity is plotted in the adjacent color bar while the C3 intensities are false-colored in red. Representative Raman spectra are also shown for cells only and cells incubated with C3 in M and N. Since the C-H region is not affected by C3, it is used to isolate cellular regions, while the Raman spectra of the CNPs are used to isolate the C3s.
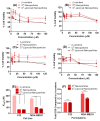
MTT assay for the evaluation of cytotoxic effects of β-carotene in free or passivated to CNPs with post coatings of amphiphilic phospholipidic assembly. Experiment was performed in (A,C) MDA-MB231 breast cancer and (B,D) C32 melanoma cells after incubation for (A,B) 48 and (C,D) 72 h at various concentrations (125, 62.5, 31.25, 15.6125, 7. 8 and 3.9 μM) of β-carotene in free or form of C3 nanoparticles and C3-Lipocoat nanoparticle formulations. (E) Comparative IC50 values of used formulations and (F) fold change across both the cell lines. Statistical analysis performed using Two-way ANOVA on IC50 values represented as *** for p < 0.001.
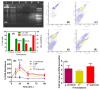
(A) DNA laddering assay performed on genomic DNA extracted from MDA-MB231 cells (lane 1) untreated; or treated with (lane 2) C3-Lipocoat; (lane 3) C3 nanoparticles; (lane 4) CNP and (lane 5) β-carotene at concentration of 100 μM. Here lane 6 represent DNA ladder of 1–10 kb. Scatter plots obtained from 4% paraformaldehyde fixed MDA-MB231 cells (B) untreated or treated with (C) β-carotene; (D) C3 and (E) C3-Lipocoat at concentration of 100 μM post propidium iodide (PI; 10 μg/mL) staining. Scattering events in R7 and R2 gates represent the cells from live cell and apoptotic cell population, respectively. (F) Scattering events revealed the increasing % of apoptotic cell population in order of β-carotene, C3 and C3-Lipocoat while % live population followed the reverse order with maximum in untreated cells. (G) Variation in ROS abundance with time of incubation and change in β-carotene formulation in MDA-MB231 with maximum fold increase by C3-Lipocoat compared to CNP itself (H). Statistical analysis performed using Two-way ANOVA on IC50 values represented as *** for p < 0.001.
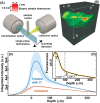
Panel A shows the 3D fluorescence experimental geometry. The objective generates a plane sheet of light that irradiates the sample. Fluorescence is acquired orthogonal to the light plane. Panel B shows a three dimensional rendering of background subtracted fluorescence images of pigskin treated with C3. Panel C shows the integrated fluorescence intensity with skin depth for both treated and untreated samples. Panel D shows the concentration profile of C3 within 2 layers of the skin upon diffusion.
Similar articles
-
Yi J, Lam TI, Yokoyama W, Cheng LW, Zhong F. Yi J, et al. J Agric Food Chem. 2014 Feb 5;62(5):1096-104. doi: 10.1021/jf404073c. Epub 2014 Jan 24. J Agric Food Chem. 2014. PMID: 24422504
-
Wu VM, Huynh E, Tang S, Uskoković V. Wu VM, et al. Acta Biomater. 2019 Apr 1;88:422-447. doi: 10.1016/j.actbio.2019.01.064. Epub 2019 Feb 1. Acta Biomater. 2019. PMID: 30711662
-
Wen Y, Xu M, Liu X, Jin X, Kang J, Xu D, Sang H, Gao P, Chen X, Zhao L. Wen Y, et al. Colloids Surf B Biointerfaces. 2019 Jan 1;173:842-850. doi: 10.1016/j.colsurfb.2018.10.073. Epub 2018 Oct 27. Colloids Surf B Biointerfaces. 2019. PMID: 30551300
-
Nanoparticle colloidal stability in cell culture media and impact on cellular interactions.
Moore TL, Rodriguez-Lorenzo L, Hirsch V, Balog S, Urban D, Jud C, Rothen-Rutishauser B, Lattuada M, Petri-Fink A. Moore TL, et al. Chem Soc Rev. 2015 Oct 7;44(17):6287-6305. doi: 10.1039/c4cs00487f. Epub 2015 Jun 9. Chem Soc Rev. 2015. PMID: 26056687 Review.
-
Quantitative uptake of colloidal particles by cell cultures.
Feliu N, Hühn J, Zyuzin MV, Ashraf S, Valdeperez D, Masood A, Said AH, Escudero A, Pelaz B, Gonzalez E, Duarte MAC, Roy S, Chakraborty I, Lim ML, Sjöqvist S, Jungebluth P, Parak WJ. Feliu N, et al. Sci Total Environ. 2016 Oct 15;568:819-828. doi: 10.1016/j.scitotenv.2016.05.213. Epub 2016 Jun 13. Sci Total Environ. 2016. PMID: 27306826 Review.
Cited by
-
Zhou Q, Liu Q, Wang Y, Chen J, Schmid O, Rehberg M, Yang L. Zhou Q, et al. Adv Sci (Weinh). 2024 Apr;11(14):e2308659. doi: 10.1002/advs.202308659. Epub 2024 Jan 28. Adv Sci (Weinh). 2024. PMID: 38282076 Free PMC article. Review.
-
Use of acidic nanoparticles to rescue macrophage lysosomal dysfunction in atherosclerosis.
Zhang X, Misra SK, Moitra P, Zhang X, Jeong SJ, Stitham J, Rodriguez-Velez A, Park A, Yeh YS, Gillanders WE, Fan D, Diwan A, Cho J, Epelman S, Lodhi IJ, Pan D, Razani B. Zhang X, et al. Autophagy. 2023 Mar;19(3):886-903. doi: 10.1080/15548627.2022.2108252. Epub 2022 Aug 18. Autophagy. 2023. PMID: 35982578 Free PMC article.
-
Byrne HJ, Bonnier F, Efeoglu E, Moore C, McIntyre J. Byrne HJ, et al. Front Bioeng Biotechnol. 2020 Oct 29;8:544311. doi: 10.3389/fbioe.2020.544311. eCollection 2020. Front Bioeng Biotechnol. 2020. PMID: 33195114 Free PMC article. Review.
-
Ortega-Regules AE, Martínez-Thomas JA, Schürenkämper-Carrillo K, de Parrodi CA, López-Mena ER, Mejía-Méndez JL, Lozada-Ramírez JD. Ortega-Regules AE, et al. Plants (Basel). 2024 Jun 7;13(12):1584. doi: 10.3390/plants13121584. Plants (Basel). 2024. PMID: 38931016 Free PMC article. Review.
-
Kampert T, Misra SK, Srivastava I, Tripathi I, Pan D. Kampert T, et al. Cell Mol Bioeng. 2017 May 23;10(5):371-386. doi: 10.1007/s12195-017-0490-y. eCollection 2017 Oct. Cell Mol Bioeng. 2017. PMID: 31719869 Free PMC article.
References
-
- Jia F. et al.. Multifunctional nanoparticles for targeted delivery of immune activating and cancer therapeutic agents. J. Control. Release 172, 1020–1034 (2013). - PubMed
-
- Sanvicens N. & Marco M. P. Multifunctional nanoparticles--properties and prospects for their use in human medicine. Trends Biotechnol. 26, 425–433 (2008). - PubMed
-
- Lee D.-E. et al.. Multifunctional nanoparticles for multimodal imaging and theragnosis. Chem. Soc. Rev. 41, 2656–2672 (2012). - PubMed
-
- Misra S. K., Kim B., Chang H.-H. & Pan D. Defying symmetry: a ‘flowery’ architecture with augmented surface area from gold coated polymeric ‘nanoblooms’. RSC Adv. 4, 34303–34306 (2014).
Publication types
MeSH terms
Substances
LinkOut - more resources
Full Text Sources
Other Literature Sources
Miscellaneous