Reference compounds for alternative test methods to indicate developmental neurotoxicity (DNT) potential of chemicals: example lists and criteria for their selection and use - PubMed
doi: 10.14573/altex.1604201. Epub 2016 Jul 25.
Sandra Ceccatelli 2 , Mardas Daneshian 3 , Ellen Fritsche 4 , Nina Hasiwa 3 , Thomas Hartung 3 5 , Helena T Hogberg 5 , Marcel Leist 3 6 7 , Abby Li 8 , William R Mundi 9 , Stephanie Padilla 9 , Aldert H Piersma 10 11 , Anna Bal-Price 12 , Andrea Seiler 13 , Remco H Westerink 14 , Bastian Zimmer 15 , Pamela J Lein 16 17
Affiliations
- PMID: 27452664
- PMCID: PMC5250586
- DOI: 10.14573/altex.1604201
Reference compounds for alternative test methods to indicate developmental neurotoxicity (DNT) potential of chemicals: example lists and criteria for their selection and use
Michael Aschner et al. ALTEX. 2017.
Abstract
There is a paucity of information concerning the developmental neurotoxicity (DNT) hazard posed by industrial and environmental chemicals. New testing approaches will most likely be based on batteries of alternative and complementary (non-animal) tests. As DNT is assumed to result from the modulation of fundamental neurodevelopmental processes (such as neuronal differentiation, precursor cell migration or neuronal network formation) by chemicals, the first generation of alternative DNT tests target these processes. The advantage of such types of assays is that they capture toxicants with multiple targets and modes-of-action. Moreover, the processes modelled by the assays can be linked to toxicity endophenotypes, i.e., alterations in neural connectivity that form the basis for neurofunctional deficits in man. The authors of this review convened in a workshop to define criteria for the selection of positive/negative controls, to prepare recommendations on their use, and to initiate the setup of a directory of reference chemicals. For initial technical optimization of tests, a set of > 50 endpoint-specific control compounds was identified. For further test development, an additional "test" set of 33 chemicals considered to act directly as bona fide DNT toxicants is proposed, and each chemical is annotated to the extent it fulfills these criteria. A tabular compilation of the original literature used to select the test set chemicals provides information on statistical procedures, and toxic/non-toxic doses (both for pups and dams). Suggestions are provided on how to use the > 100 compounds (including negative controls) compiled here to address specificity, adversity and use of alternative test systems.
Keywords: AOP; neurotoxicity; specificity; test development; validation.
Conflict of interest statement
The authors declare that they have no conflicts of interest.
Figures
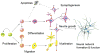
Several fundamental neurodevelopmental processes are absolutely necessary for nervous system development, and therefore well-conserved across species. Moreover, the processes known from in vivo studies can be relatively faithfully modeled in vitro. It is assumend that DNT exert their toxicity, because they disturb at least one of these processes. Therefore, disturbances of the processes depicted here are KE of AOP relevant for DNT.
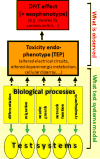
For development of relevant model systems, we need approaches for linking the observable DNT effect (= exophenotype; see red box) triggered by a xenobiotic to effects that this compound has in in vitro test systems (yellow circles). Toxicity endophenotypes (orange box) form the conceptual link between what is observed in man or experimental animals and on what test systems model. They are a description of the altered biological state of the nervous system (e.g. neuronal disarray in the frontal cortex) in vivo that causes the externally observable DNT phenotype (e.g. reduced IQ). Thus, ‘toxicity endophenotypes (TEP)’ describe the altered functional or structural connectivity or responsiveness of parts of the nervous system, triggered by xenobiotics. The TEP results from the disturbance of one or several fundamental biological processes (e.g. neurite growth). Notably, there may be a delay or lag of years between disturbance of a process by a chemical and the observation of DNT effects (dashed arrows linking processes and TEP). Both the setup of model systems and the characterization of tool compounds to validate such systems requires that we establish the following connections: (1) exophenotype to TEP (the exophenotype is the only robust and relevant starting point for identification of DNT compounds known at present); (2) association of TEP with disturbed biological process(es) that led to the TEP; (3) link of in vitro test system endpoint to prediction of a disturbed biological process in vivo. The fundamental biological processes as such (but not the TEP) may be modeled by alternative test systems. Thus, the test systems are inspired by the biological processes (green arrows), but the outcome of test systems predicts to some extent certain TEP (e.g. inhibited neuronal migration predicts neuronal disarray and/or a deficit in neuronal number in some brain region). In this sense, TEP represent the level of organisation that links in vitro test systems for fundamental biological processes to apical DNT endpoints (exophenotypes).
Similar articles
-
Crofton K, Fritsche E, Ylikomi T, Bal-Price A. Crofton K, et al. ALTEX. 2014;31(2):223-4. doi: 10.14573/altex.1402121. ALTEX. 2014. PMID: 24794006 No abstract available.
-
Human Ntera2 cells as a predictive in vitro test system for developmental neurotoxicity.
Stern M, Gierse A, Tan S, Bicker G. Stern M, et al. Arch Toxicol. 2014 Jan;88(1):127-36. doi: 10.1007/s00204-013-1098-1. Epub 2013 Aug 7. Arch Toxicol. 2014. PMID: 23917397
-
Crofton KM, Mundy WR, Lein PJ, Bal-Price A, Coecke S, Seiler AE, Knaut H, Buzanska L, Goldberg A. Crofton KM, et al. ALTEX. 2011;28(1):9-15. ALTEX. 2011. PMID: 21311847
-
Developmental neurotoxicity test guidelines: problems and perspectives.
Tohyama C. Tohyama C. J Toxicol Sci. 2016;41(Spec):SP69-SP79. doi: 10.2131/jts.41.SP69. J Toxicol Sci. 2016. PMID: 28250285 Review.
-
Expanding the test set: Chemicals with potential to disrupt mammalian brain development.
Mundy WR, Padilla S, Breier JM, Crofton KM, Gilbert ME, Herr DW, Jensen KF, Radio NM, Raffaele KC, Schumacher K, Shafer TJ, Cowden J. Mundy WR, et al. Neurotoxicol Teratol. 2015 Nov-Dec;52(Pt A):25-35. doi: 10.1016/j.ntt.2015.10.001. Epub 2015 Oct 22. Neurotoxicol Teratol. 2015. PMID: 26476195 Review.
Cited by
-
Hogberg HT, de Cássia da Silveira E Sá R, Kleensang A, Bouhifd M, Cemiloglu Ulker O, Smirnova L, Behl M, Maertens A, Zhao L, Hartung T. Hogberg HT, et al. Arch Toxicol. 2021 Jan;95(1):207-228. doi: 10.1007/s00204-020-02903-2. Epub 2020 Oct 19. Arch Toxicol. 2021. PMID: 33078273 Free PMC article.
-
Dach K, Yaghoobi B, Schmuck MR, Carty DR, Morales KM, Lein PJ. Dach K, et al. Toxicol Sci. 2019 Jan 1;167(1):77-91. doi: 10.1093/toxsci/kfy266. Toxicol Sci. 2019. PMID: 30364989 Free PMC article.
-
Heavy Metal Neurotoxicants Induce ALS-Linked TDP-43 Pathology.
Ash PEA, Dhawan U, Boudeau S, Lei S, Carlomagno Y, Knobel M, Al Mohanna LFA, Boomhower SR, Newland MC, Sherr DH, Wolozin B. Ash PEA, et al. Toxicol Sci. 2019 Jan 1;167(1):105-115. doi: 10.1093/toxsci/kfy267. Toxicol Sci. 2019. PMID: 30371865 Free PMC article.
-
Lee J, Schlichting R, König M, Scholz S, Krauss M, Escher BI. Lee J, et al. ACS Environ Au. 2022 Aug 17;2(6):523-535. doi: 10.1021/acsenvironau.2c00026. eCollection 2022 Nov 16. ACS Environ Au. 2022. PMID: 37101724 Free PMC article.
-
Blood-brain barrier development: Systems modeling and predictive toxicology.
Saili KS, Zurlinden TJ, Schwab AJ, Silvin A, Baker NC, Hunter ES 3rd, Ginhoux F, Knudsen TB. Saili KS, et al. Birth Defects Res. 2017 Dec 1;109(20):1680-1710. doi: 10.1002/bdr2.1180. Birth Defects Res. 2017. PMID: 29251840 Free PMC article. Review.
References
-
- Albright TD, Jessell TM, Kandel ER, et al. Neural science: a century of progress and the mysteries that remain. Neuron. 2000;25(Suppl):S1–55. http://dx.doi.org/10.1016/s0896-6273(00)80912-5. - DOI - PubMed
-
- Alepee N, Bahinski A, Daneshian M, et al. State-of-the-art of 3D cultures (organs-on-a-chip) in safety testing and pathophysiology. ALTEX. 2014;31:441–477. http://dx.doi.org/http://dx.doi.org/10.14573/altex1406111. - DOI - PMC - PubMed
-
- Ali MM, Murthy RC, Chandra SV. Developmental and longterm neurobehavioral toxicity of low level in-utero cadmium exposure in rats. Neurobehav Toxicol Teratol. 1986;8:463–468. - PubMed
-
- Ankley GT, Bennett RS, Erickson RJ, et al. Adverse outcome pathways: a conceptual framework to support ecotoxicology research and risk assessment. Environ Toxicol Chem. 2010;29:730–741. http://dx.doi.org/10.1002/etc.34. - DOI - PubMed
-
- Asimiadou S, Bittigau P, Felderhoff-Mueser U, et al. Protection with estradiol in developmental models of apoptotic neurodegeneration. Ann Neurol. 2005;58:266–276. http://dx.doi.org/10.1002/ana.20553. - DOI - PubMed
MeSH terms
Substances
Grants and funding
LinkOut - more resources
Full Text Sources
Other Literature Sources
Research Materials