RhoA inhibits neural differentiation in murine stem cells through multiple mechanisms - PubMed
- ️Fri Jan 01 2016
RhoA inhibits neural differentiation in murine stem cells through multiple mechanisms
Junning Yang et al. Sci Signal. 2016.
Abstract
Spontaneous neural differentiation of embryonic stem cells is induced by Noggin-mediated inhibition of bone morphogenetic protein 4 (BMP4) signaling. RhoA is a guanosine triphosphatase (GTPase) that regulates cytoskeletal dynamics and gene expression, both of which control stem cell fate. We found that disruption of Syx, a gene encoding a RhoA-specific guanine nucleotide exchange factor, accelerated retinoic acid-induced neural differentiation in murine embryonic stem cells aggregated into embryoid bodies. Cells from Syx(+/+) and Syx(-/-) embryoid bodies had different abundances of proteins implicated in stem cell pluripotency. The differentiation-promoting proteins Noggin and RARγ (a retinoic acid receptor) were more abundant in cells of Syx(-/-) embryoid bodies, whereas the differentiation-suppressing proteins SIRT1 (a protein deacetylase) and the phosphorylated form of SMAD1 (the active form of this transcription factor) were more abundant in cells of Syx(+/+) embryoid bodies. These differences were blocked by the overexpression of constitutively active RhoA, indicating that the abundance of these proteins was maintained, at least in part, by RhoA activity. The peripheral stress fibers in cells from Syx(-/-) embryoid bodies were thinner than those in Syx(+/+) cells. Furthermore, less Noggin and fewer vesicles containing Rab3d, a GTPase that mediates Noggin trafficking, were detected in cells from Syx(-/-) embryoid bodies, which could result from increased Noggin exocytosis. These results suggested that, in addition to inhibiting Noggin transcription, RhoA activity in wild-type murine embryonic stem cells also prevented neural differentiation by limiting Noggin secretion.
Copyright © 2016, American Association for the Advancement of Science.
Figures
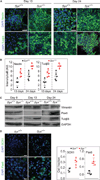
(A) Immunofluorescence images of nestin- or Tubβ3-labeled cells that were dissociated from RA-treated Syx+/+ and Syx−/− EBs at the indicated times (scale bars, 50 µm). DAPI, 4′,6-diamidino-2-phenylindole. (B) Histograms quantifying nestin and Tubβ3 immunofluorescence intensities shown in (A) [two-way analysis of variance (ANOVA), n = 5 fields, each containing >90 Syx+/+ cells or >130 Syx−/− cells, acquired in two independent experiments; mean ± SD, P < 0.001 for all differences]. AU, arbitrary units. (C) Immunoblot of neural differentiation markers vimentin, Pax6, and Tubβ3 in one of two independent experiments with cells dissociated from RA-treated Syx+/+ and Syx−/− EBs at the indicated time points. GAPDH (glyceraldehyde-3-phosphate dehydrogenase) was used as a loading control. (D) Immunofluorescence images of the neural progenitor cell markers SOX1 and Pax6 in cells from RA-treated Syx+/+ and Syx−/− EBs 12 days after EB aggregation (scale bars, 50 µm). Quantification of the immunofluorescence of each marker is shown in the adjoining histograms [n = 5 fields containing 138 (Pax6, Syx+/+), 82 (Pax6, Syx−/−), 122 (SOX1, Syx+/+), and 93 (SOX1, Syx−/−) cells from two independent experiments; Pax6: 26.2 higher odds for the presence of Pax6 in Syx−/− rather than in Syx+/+ cells (95% confidence interval, 10.6 to 64.8; P < 0.001); SOX1: 2.4 higher odds for the presence of SOX1 in Syx−/− rather than in Syx+/+ cells (95% confidence interval, 1.6 to 3.4; P < 0.001)].
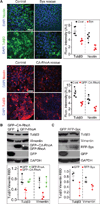
(A) Immunofluorescence images of cells dissociated from RA-treated Syx−/− EBs, transfected with RFP-Syx or GFP–CA-RhoA, and immunolabeled as indicated. GFP was used as a negative control. The adjoining histograms show the quantification of Tubβ3 and nestin immunofluorescence in images of cells from each group (two-sample t test, equal variance, n = 5 fields from three independent experiments, each containing >130 cells, mean ± SD; Syx rescue: P = 0.003 for Tubβ3, P < 0.001 for nestin; CA-RhoA rescue: P = 0.012 for Tubβ3, P < 0.001 for nestin). (B) Immunoblot of the neural differentiation markers Tubβ3 and nestin in cells from RA-treated Syx+/+ EBs transfected with GFP, GFP-RhoA, or GFP–CA-RhoA. The histogram shows the quantification of Tubβ3 and vimentin band densities normalized to GAPDH band density in cells transfected with either Syx or CA-RhoA (one-way ANOVA, n = 3 independent experiments, mean ± SD; Tubβ3: P = 0.015 for overall difference between the three groups, P = 0.008 for GFP–CA-RhoA to GFP, P = 0.0013 for GFP– CA-RhoA to GFP-RhoA; vimentin: P = 0.013 for overall difference between the three groups, P = 0.009 for GFP–CA-RhoA to GFP, P = 0.009 for GFP– CA-RhoA to GFP-RhoA). RBD, relative band density. (C) As in (B), in Syx−/− cells rescued by GFP or by RFP-Syx (two-sample t test, equal variance, n = 3 independent experiments, mean ± SD; P = 0.048 for Tubβ3, P = 0.043 for vimentin). GAPDH is a loading control.
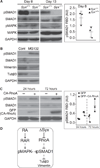
(A) Immunoblots showing the phosphorylation of SMAD1 (pSMAD1) and MAPK (pMAPK) in cells dissociated from RA-treated Syx+/+ or Syx−/− EBs at the indicated times (two-sample t test, equal variance, n = 3 independent experiments, mean ± SD, P = 0.049). GAPDH is a loading control. (B) Cells dissociated from RA-treated Syx+/+ or Syx−/− EBs 4 days after aggregation were treated with MG132 to inhibit proteasomal degradation or with dimethyl sulfoxide as a negative control (Cont) followed by immunoblotting with the indicated antibodies (one of two independent experiments). (C) Immunoblots of cells from RA-treated Syx−/− EBs transfected with GFP–CA-RhoA and probed at the indicated times to detect pSMAD1 (two-sample t test, equal variance, n = 3 independent experiments, mean ± SD, P = 0.0095 for 24 hours, P = 0.046 for 72 hours). (D) Signaling scheme representing the data shown in (A) to (C). Solid lines represent direct regulatory events, whereas dashed lines represent events that either are indirect or have not been shown to be direct.
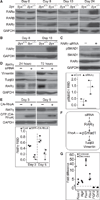
(A) Immunoblot of RARs at the indicated times in RA-treated Syx+/+ and Syx−/− EBs (one of two independent experiments). GAPDH is a loading control. (B) Immunoblot showing RARγ production at day 13 in Syx−/− EBs in the absence of RA (one of two independent experiments). (C) Immunoblot showing phosphorylated SMAD1 (pSMAD1) after RARγ knockdown in cells dissociated from RA-treated Syx−/− EBs. Quantification of normalized pSMAD1 band density is shown in the histogram (two-sample t test, unequal variance, n = 3 independent experiments, mean ± SD, P = 0.047). (D). Immunoblot showing markers of neural differentiation vimentin and Tubβ3 after RARγ knockdown in cells dissociated from RA-treated Syx−/− EBs (one of two independent experiments). (E) Immunoblot showing RARγ in cells dissociated from Syx−/− EBs at the indicated times and transfected with GFP–CA-RhoA. Quantification is shown in the histogram (two-way ANOVA, n = 3 independent experiments, mean ± SD, P < 0.001 for both times). (F) Signaling scheme representing the data shown in (A) to (E). Solid lines represent direct regulatory events, whereas dashed lines represent events that either are indirect or have not been shown to be direct. (G) Quantitative real-time polymerase chain reaction (qRT-PCR) measurements of mRNA abundances of the indicated genes in Syx−/− relative to Syx+/+ cells from 13-day-old RA-naïve EBs (n = 3 replicates).
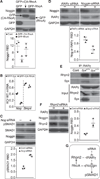
(A) Transfection of cells from RA-treated Syx−/− EBs with GFP–CA-RhoA, but not GFP-RhoA, reduced Noggin production (one-way ANOVA, n = 3 independent experiments, mean ± SD, P = 0.018 for GFP to CA-RhoA). GAPDH is a loading control. (B) qRT-PCR results showing the effects of Syx or CA-RhoA transfection on the relative abundances of Noggin (Nog) and Bmp4 in cells from RA-treated Syx+/+ and Syx−/− EBs (two-sample t test, equal variance, mean ± SD, n = 3 independent experiments, P < 0.001 for Nog, P = 0.045 for Bmp4). (C) Immunoblot showing that Noggin knockdown increased SMAD1 phosphorylation (pSMAD1) in cells from RA-treated EBs (two-sample t test, unequal variance, mean ± SD, n = 3 independent experiments, P < 0.001). (D) Immunoblot showing that Rarγ silencing in cells from RA-treated EBs reduced Noggin production, but Nog silencing did not reduce RARγ production (two-sample t test, unequal variance, mean ± SD, n = 3 independent experiments, P = 0.047). (E) RARγ coimmunoprecipitated with RHPN2 and Syx in extracts from mESCs (one of two independent experiments). (F) Immunoblot showing RARγ, Noggin, and RHPN2 after transfection of cells from RA-treated EBs with siRNA targeting RHPN2 or a control siRNA (two-sample t test, unequal variance, n = 3 three independent experiments, mean ± SD, P = 0.041). (G) Signaling scheme representing the data shown in (A), (C), and (D). Solid lines represent direct regulatory events, whereas dashed lines represent events that either are indirect or have not been shown to be direct.
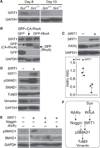
(A) Immunoblot showing SIRT1 abundance in dissociated Syx+/+ or Syx−/− EBs at the indicated time points (one of two independent experiments). GAPDH is a loading control. (B) Immunoblot of cells from dissociated RA-treated Syx−/− EBs after transfection by GFP–CA-RhoA and GFP–wild-type RhoA (one of two independent experiments). (C) Immunoblot showing the effect of SIRT1 transfection on RARγ production in cells dissociated from RA-treated EBs (two-sample t test, equal variance, mean ± SD, n = 3 independent experiments, P = 0.01). (D) Immunoblot showing that transfection of cells dissociated from RA-treated Syx+/+ EBs with SIRT1 increased SMAD1 phosphorylation (pSMAD1) and reduced the abundance of the neural differentiation markers Tubβ3 and vimentin (one of two independent experiments). (E) Effect of SIRT1 coexpression with Noggin or Rarγ on SMAD1 phosphorylation in 8-day EBs transfected by the indicated plasmids (one of two independent experiments; the left lanes were immunoblotted on a separate membrane). (F) Signaling scheme representing the data shown in (A) to (E). Solid lines represent direct regulatory events, whereas dashed lines represent events that either are indirect or have not been shown to be direct.
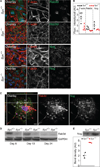
(A) Immunofluorescence of F-actin and the vesicular marker Rab3d or Noggin (Nog) in cells dissociated from RA-treated EBs (scale bars, 25 µm). (B) Quantification of F-actin, Rab3d, and Noggin immunofluorescence intensity per cell in cells from RA-treated Syx+/+ or Syx−/− EBs (two-sample t test, unequal variance, mean ± SD, n = 5 fields from two independent experiments, 35 to 50 cells per field; P = 0.008 for F-actin, P = 0.007 for Rab3d, P = 0.017 for Noggin). (C) Immunofluorescence image of a Syx+/+ mESC showing colocalization of FLAG-tagged Rab3d with endogenous Noggin in cytoplasmic punctae in the focal plane (arrowheads) and in optical sections along the white lines (one of two independent experiments). (D) Immunoblot of Rab3d in cells from RA-treated Syx+/+ and Syx−/− EBs at the indicted time points (one of two independent experiments). GAPDH is a loading control. (E) Immunoblot of Noggin in the medium of cells from RA-treated Syx+/+ and Syx−/− EBs (two-sample t test, unequal variance, mean ± SD, n = 3 independent experiments, P = 0.015).
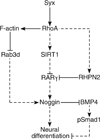
Solid lines represent direct regulatory events, whereas dashed lines represent events that either are indirect or have not been shown to be direct.
Similar articles
-
The versatility of RhoA activities in neural differentiation.
Horowitz A, Yang J, Cai J, Iacovitti L. Horowitz A, et al. Small GTPases. 2019 Jan;10(1):26-32. doi: 10.1080/21541248.2016.1273171. Epub 2017 Jan 27. Small GTPases. 2019. PMID: 28125332 Free PMC article.
-
The RhoA GEF Syx is a target of Rnd3 and regulated via a Raf1-like ubiquitin-related domain.
Goh LL, Manser E. Goh LL, et al. PLoS One. 2010 Aug 25;5(8):e12409. doi: 10.1371/journal.pone.0012409. PLoS One. 2010. PMID: 20811643 Free PMC article.
-
Rab13-dependent trafficking of RhoA is required for directional migration and angiogenesis.
Wu C, Agrawal S, Vasanji A, Drazba J, Sarkaria S, Xie J, Welch CM, Liu M, Anand-Apte B, Horowitz A. Wu C, et al. J Biol Chem. 2011 Jul 1;286(26):23511-20. doi: 10.1074/jbc.M111.245209. Epub 2011 May 4. J Biol Chem. 2011. PMID: 21543326 Free PMC article.
-
Ernkvist M, Luna Persson N, Audebert S, Lecine P, Sinha I, Liu M, Schlueter M, Horowitz A, Aase K, Weide T, Borg JP, Majumdar A, Holmgren L. Ernkvist M, et al. Blood. 2009 Jan 1;113(1):244-53. doi: 10.1182/blood-2008-04-153874. Epub 2008 Sep 29. Blood. 2009. PMID: 18824598 Free PMC article.
-
NRP-1 interacts with GIPC1 and SYX to activate p38 MAPK signaling and cancer stem cell survival.
Grun D, Adhikary G, Eckert RL. Grun D, et al. Mol Carcinog. 2019 Apr;58(4):488-499. doi: 10.1002/mc.22943. Epub 2018 Dec 21. Mol Carcinog. 2019. PMID: 30456845 Free PMC article.
Cited by
-
The versatility of RhoA activities in neural differentiation.
Horowitz A, Yang J, Cai J, Iacovitti L. Horowitz A, et al. Small GTPases. 2019 Jan;10(1):26-32. doi: 10.1080/21541248.2016.1273171. Epub 2017 Jan 27. Small GTPases. 2019. PMID: 28125332 Free PMC article.
-
Scientific Validation of Human Neurosphere Assays for Developmental Neurotoxicity Evaluation.
Koch K, Bartmann K, Hartmann J, Kapr J, Klose J, Kuchovská E, Pahl M, Schlüppmann K, Zühr E, Fritsche E. Koch K, et al. Front Toxicol. 2022 Mar 2;4:816370. doi: 10.3389/ftox.2022.816370. eCollection 2022. Front Toxicol. 2022. PMID: 35295221 Free PMC article.
-
Lin WH, Feathers RW, Cooper LM, Lewis-Tuffin LJ, Chen J, Sarkaria JN, Anastasiadis PZ. Lin WH, et al. JCI Insight. 2023 Jul 10;8(13):e157491. doi: 10.1172/jci.insight.157491. JCI Insight. 2023. PMID: 37427593 Free PMC article.
-
Yang J, Wu C, Stefanescu I, Horowitz A. Yang J, et al. J Vis Exp. 2017 Apr 22;(122):55621. doi: 10.3791/55621. J Vis Exp. 2017. PMID: 28518115 Free PMC article.
-
Oh B, Wu YW, Swaminathan V, Lam V, Ding J, George PM. Oh B, et al. Adv Sci (Weinh). 2021 Feb 18;8(7):2002112. doi: 10.1002/advs.202002112. eCollection 2021 Apr. Adv Sci (Weinh). 2021. PMID: 33854874 Free PMC article.
References
-
- Chen X, Xu H, Yuan P, Fang F, Huss M, Vega VB, Wong E, Orlov YL, Zhang W, Jiang J, Loh Y-H, Yeo HC, Yeo ZX, Narang V, Govindarajan KR, Leong B, Shahab A, Ruan Y, Bourque G, Sung W-K, Clarke ND, Wei C-L, Ng H-H. Integration of external signaling pathways with the core transcriptional network in embryonic stem cells. Cell. 2008;133:1106–1117. - PubMed
-
- Liang J, Wan M, Zhang Y, Gu P, Xin H, Jung SY, Qin J, Wong J, Cooney AJ, Liu D, Songyang Z. Nanog and Oct4 associate with unique transcriptional repression complexes in embryonic stem cells. Nat. Cell Biol. 2008;10:731–739. - PubMed
-
- Loh Y-H, Wu Q, Chew J-L, Vega VB, Zhang W, Chen X, Bourque G, George J, Leong B, Liu J, Wong K-Y, Sung KW, Lee CWH, Zhao X-D, Chiu K-P, Lipovich L, Kuznetsov VA, Robson P, Stanton LW, Wei C-L, Ruan Y, Lim B, Ng H-H. The Oct4 and Nanog transcription network regulates pluripotency in mouse embryonic stem cells. Nat. Genet. 2006;38:431–440. - PubMed
-
- Pan G, Thomson JA. Nanog and transcriptional networks in embryonic stem cell pluripotency. Cell Res. 2007;17:42–49. - PubMed
MeSH terms
Substances
LinkOut - more resources
Full Text Sources
Other Literature Sources
Molecular Biology Databases