The A-Kinase Anchoring Protein (AKAP) Glycogen Synthase Kinase 3β Interaction Protein (GSKIP) Regulates β-Catenin through Its Interactions with Both Protein Kinase A (PKA) and GSK3β - PubMed
- ️Fri Jan 01 2016
The A-Kinase Anchoring Protein (AKAP) Glycogen Synthase Kinase 3β Interaction Protein (GSKIP) Regulates β-Catenin through Its Interactions with Both Protein Kinase A (PKA) and GSK3β
Alessandro Dema et al. J Biol Chem. 2016.
Abstract
The A-kinase anchoring protein (AKAP) GSK3β interaction protein (GSKIP) is a cytosolic scaffolding protein binding protein kinase A (PKA) and glycogen synthase kinase 3β (GSK3β). Here we show that both the AKAP function of GSKIP, i.e. its direct interaction with PKA, and its direct interaction with GSK3β are required for the regulation of β-catenin and thus Wnt signaling. A cytoplasmic destruction complex targets β-catenin for degradation and thus prevents Wnt signaling. Wnt signals cause β-catenin accumulation and translocation into the nucleus, where it induces Wnt target gene expression. GSKIP facilitates control of the β-catenin stabilizing phosphorylation at Ser-675 by PKA. Its interaction with GSK3β facilitates control of the destabilizing phosphorylation of β-catenin at Ser-33/Ser-37/Thr-41. The influence of GSKIP on β-catenin is explained by its scavenger function; it recruits the kinases away from the destruction complex without forming a complex with β-catenin. The regulation of β-catenin by GSKIP is specific for this AKAP as AKAP220, which also binds PKA and GSK3β, did not affect Wnt signaling. We find that the binding domain of AKAP220 for GSK3β is a conserved GSK3β interaction domain (GID), which is also present in GSKIP. Our findings highlight an essential compartmentalization of both PKA and GSK3β by GSKIP, and ascribe a function to a cytosolic AKAP-PKA interaction as a regulatory factor in the control of canonical Wnt signaling. Wnt signaling controls different biological processes, including embryonic development, cell cycle progression, glycogen metabolism, and immune regulation; deregulation is associated with diseases such as cancer, type 2 diabetes, inflammatory, and Alzheimer's and Parkinson's diseases.
Keywords: A-kinase anchoring protein (AKAP); GSKIP; Wnt signaling; beta-catenin (β-catenin); cell compartmentalization; glycogen synthase kinase 3 (GSK-3); glycogen synthase kinase 3β interaction protein; protein kinase.
© 2016 by The American Society for Biochemistry and Molecular Biology, Inc.
Figures
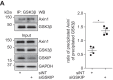
GSKIP recruits GSK3β from Axin. HEK293 cells were treated with siRNA to knock down the expression of GSKIP (siGSKIP) or with control non-targeting siRNA (siNT). GSK3β was immunoprecipitated, and GSK3β and co-immunoprecipitated Axin1 were detected by Western blotting (WB). Signals were semi-quantitatively analyzed by densitometry, and the ratio of precipitated Axin1 to GSK3β was calculated; n = 4, mean ± S.E., ANOVA, *, p < 0.05.
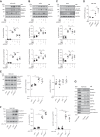
Endogenous GSKIP enhances Wnt signaling through controlling PKA phosphorylation of β-catenin at Ser-675. HEK293, A549, and HeLa cells were left untreated or treated as indicated with siRNA to knock down GSKIP (siGSKIP), control siRNA (siNT), Wnt3a-enriched medium, or control medium in the absence or presence of the PKA inhibitors, PKA inhibitor peptide (PKI) or H89, or the proteasome inhibitor MG-132. A–C, cytosol fractions were purified from the three cell types and analyzed by Western blotting (WB) for expression of the indicated proteins. The signals were densitometrically analyzed, and ratios of β-catenin/GAPDH and Ser(p)675-β-catenin/GAPDH were calculated; n ≥ 6, mean ± S.E., ANOVA, **, p < 0.01, ***, p < 0.001. D, TOPflash/FOPflash (TOP/FOP) luciferase assay. HEK293 cells were stimulated with Wnt-enriched or control medium; n = 4, mean ± S.E., ANOVA, **, p < 0.01. E, cell lysates were purified from HEK293 cells, and the indicated proteins were detected by Western blotting. Semi-quantitative densitometric analysis of Western blots is depicted as ratios of Ser(P)1490 LRP6/GAPDH and Ser(P)9 GSK3β/GAPDH; n = 6, mean ± S.E., ANOVA, *, p < 0.05, ***, p < 0.001. F, cytosolic fractions were purified, and the indicated proteins were detected by Western blotting. Semi-quantitative densitometric analysis of Western blots shows the ratios of β-catenin/GAPDH and (Ser(P)33/Ser-37/Thr-41)-β-catenin/GAPDH; n = 6, mean ± S.E., ANOVA, ***, p < 0.001. pS33/S37/T41, Ser(P)33/Ser-37/Thr-41. G, the enrichment of the indicated proteins in the cytosolic fractions of HEK293 cells that were used in A was confirmed by Western blotting. The cells were treated with Wnt3a-enriched or control medium. Shown are representative blots from n ≥ 4 experiments.
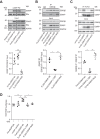
Elevated levels of GSKIP cause up-regulation of Wnt signaling under resting conditions through interactions with both PKA and GSK3β. A, HEK293 cells transiently expressing an empty control vector or the indicated GSKIP variants were subjected to cAMP-agarose precipitations. GSKIP, regulatory RIIα subunits of PKA, and HSP90 (as a control) were detected by Western blotting (WB). Semi-quantitative densitometric analysis of results is shown as the ratio of the GSKIP pulldown (PD) to GSKIP in the input. The interaction of regulatory RIIα subunits of PKA with GSKIP-N42I is strongly reduced, but not that with the GSK3β-binding-deficient mutant CFP-GSKIP-L130P; n = 5, mean ± S.E., ANOVA, **, p < 0.01. B and C, endogenous GSK3β (B) or FLAG-tagged GSKIP versions (C) were immunoprecipitated (IP; IgG, negative control immunoprecipitation). Precipitated proteins were detected by Western blotting, and signals were analyzed by semi-quantitative densitometry. Depicted are the ratios of the GSKIP IP to the GSK3β IP × GSKIP input (B) and of the GSK3β IP to the GSKIP IP (C); n = 3, mean ± S.E., ANOVA, *, p < 0.05, **, p < 0.01. D, TOPflash/FOPflash (TOP/FOP) luciferase assays using HEK293 cells expressing the indicated proteins in the absence of a Wnt signal (Wnt; n = 4, mean ± S.E., ANOVA, *, p < 0.05, **, p < 0.01.
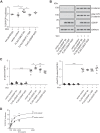
The interaction of GSKIP with both GSK3β and PKA is required for enhancing Wnt signaling in the presence of a Wnt stimulus. A, HEK293 cells expressing the indicated proteins were subjected to TOPflash/FOPflash (TOP/FOP) luciferase assays to detect Wnt signaling activity in the presence of Wnt-conditioned medium. n = 4, mean ± S.E., ANOVA, *, p < 0.05. B, cytosolic fractions were purified, and the indicated proteins were detected by Western blotting. C, the signals were semi-quantitatively analyzed by densitometry; n = 5, mean ± S.E., ANOVA, *, p < 0.05, **, p < 0.01, ***, p < 0.001. D, HEK293 cells expressing an empty vector control or wild type CFP-GSKIP were stimulated with increasing amounts of recombinant Wnt3a, and TOPflash/FOPflash luciferase assays were performed to determine Wnt signaling activity; n = 4, mean ± S.E., ANOVA, ***, p < 0.001.
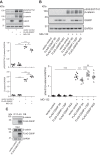
Elevated levels of GSKIP enhance Wnt signaling through controlling the GSK3β-dependent phosphorylation of β-catenin at Ser-33/Ser-37/Thr-41. A, HEK293 cells expressing an empty vector control or wild type FLAG-GSKIP were left untreated or treated with the proteasome inhibitor, MG-132. Cytosolic fractions were purified, and the indicated proteins were detected by Western blotting. Semi-quantitative densitometric analyses of the signals depict the ratios of β-catenin to GAPDH and of (Ser(P)-33/Ser-37/Thr-41)-β-catenin to GAPDH; n = 5, mean ± S.E., ANOVA, **, p < 0.01, ***, p < 0.001. pS33/S37/T41, Ser(P)-33/Ser-37/Thr-41. B, HEK293 cells expressing an empty vector control or the indicated FLAG-GSKIP variants were left untreated or treated with MG-132, and cytosolic fractions were purified and subjected to Western blotting. Semi-quantitative densitometric analysis of the signals is depicted as the ratio of (Ser(P)-33/Ser-37/Thr-41)-β-catenin to GAPDH; n = 8, mean ± S.E., ANOVA, **, p < 0.01, ***, p < 0.001. C, GSKIP and β-catenin do not form a complex in cells. HEK293 cells expressing FLAG-GSKIP or an empty control vector were subjected to an immunoprecipitation using anti-FLAG antibody. Precipitated FLAG-GSKIP and endogenous β-catenin were detected by Western blotting (WB).
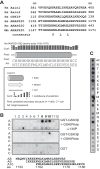
GSK3β binds AKAP220 at a conserved GID. A, alignment of the GIDs from the indicated human (Hs), rat (Rn), and mouse (Mm) proteins, and prediction of AKAP220 three-dimensional structure in the 1162–1176 region through secondary structure analysis using PSIPRED at the University College London (UCL) Department Of Computer Science. aa, amino acid. B, amino acid residues 1017–1386 of AKAP220 were spot-synthesized as 25-mer overlapping peptides and overlaid with GST alone or with GST-GSK3β that was pre-incubated with either the inactive control peptide GSKIPtide-L130P or the peptide GSKIPtide, which blocks the interaction of GSK3β with spot-synthesized peptides. Interaction of GSK3β with peptides was detected using anti-GSK3β antibodies. The sequences of the GSK3β-binding peptides in positions A8–A10 of the peptide array are shown. The sequence common in all three peptides is highlighted. C, the GID of AKAP220, amino acids 1155–1179, was spot-synthesized as 25-mer peptides (wild type (wt)) or as peptides where each amino acid was substituted by an alanine. The peptides were overlaid with recombinant GSK3β, and interaction was detected using anti-GSK3β antibodies.
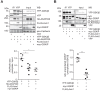
GSKIP, Axin1, Axin2, and AKAP220 compete for GSK3β binding. A, immunoprecipitation of YFP-GSK3β from HEK293 cells and detection of the indicated proteins by Western blotting (WB). The semi-quantitative analysis of co-immunoprecipitated HA-AKAP220 is depicted as the ratio of precipitated HA-AKAP220 to HA-AKAP220 in the input; data are normalized to cells expressing YFP-GSK3β and HA-AKAP220; n = 6, mean ± S.E., ANOVA, *, p < 0.05. B, co-immunoprecipitation of YFP-GSK3β and the indicated proteins from HEK293 cells. The proteins were detected by Western blotting. Semi-quantitative densitometric analysis of the signals is depicted as the ratio of precipitated GSKIP to precipitated GSK3β; n = 5, mean ± S.E., ANOVA, **, p < 0.01.
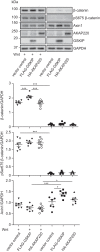
GSKIP but not AKAP220 elevates the level of β-catenin in the cytosol in response to a Wnt signal. HEK293 cells expressing the wild type GSKIP or AKAP220 or an empty vector control were stimulated with control or Wnt3a-enriched medium, cytosolic fractions were prepared, and the indicated proteins were detected by Western blotting. The signals were semi-quantitatively analyzed by densitometry and depicted as ratios; n = 7, mean ± S.E., ANOVA, *, p < 0.05, ***, p < 0.001. pS675, Ser(P)-675.
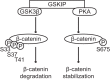
Model of GSKIP-directed regulation of β-catenin and thus of Wnt signaling. GSKIP directly binds GSK3β and PKA. It controls the stability of β-catenin and thus its transcriptional activity through facilitating its phosphorylation (indicated by circled P) by the two kinases. GSK3β phosphorylates Ser-33/Ser-37/Thr-41 in the cytoplasm and targets it for proteasomal degradation. The phosphorylation of Ser-675 by PKA stabilizes β-catenin and enhances Wnt-induced signaling.
Similar articles
-
The origin of GSKIP, a multifaceted regulatory factor in the mammalian Wnt pathway.
Chou CH, Yang MC, Hsiao BX, Wang YH, Liu HF, Chiou SJ, Chuang YC, Yang CN, Lieu AS, Loh JK, Howng SL, Chou AK, Tseng CN, Cheng JT, Hong YR. Chou CH, et al. Biochim Biophys Acta Mol Cell Res. 2018 Aug;1865(8):1046-1059. doi: 10.1016/j.bbamcr.2018.04.008. Epub 2018 Apr 23. Biochim Biophys Acta Mol Cell Res. 2018. PMID: 29694914
-
Whiting JL, Nygren PJ, Tunquist BJ, Langeberg LK, Seternes OM, Scott JD. Whiting JL, et al. J Biol Chem. 2015 Aug 7;290(32):19445-57. doi: 10.1074/jbc.M115.654822. Epub 2015 Jun 18. J Biol Chem. 2015. PMID: 26088133 Free PMC article.
-
Glycogen synthase kinase 3beta interaction protein functions as an A-kinase anchoring protein.
Hundsrucker C, Skroblin P, Christian F, Zenn HM, Popara V, Joshi M, Eichhorst J, Wiesner B, Herberg FW, Reif B, Rosenthal W, Klussmann E. Hundsrucker C, et al. J Biol Chem. 2010 Feb 19;285(8):5507-21. doi: 10.1074/jbc.M109.047944. Epub 2009 Dec 11. J Biol Chem. 2010. PMID: 20007971 Free PMC article.
-
Many faces and functions of GSKIP: a temporospatial regulation view.
Wu NS, Lin YF, Ma IC, Ko HJ, Hong YR. Wu NS, et al. Cell Signal. 2022 Sep;97:110391. doi: 10.1016/j.cellsig.2022.110391. Epub 2022 Jun 18. Cell Signal. 2022. PMID: 35728705 Review.
-
Li Q, Sun M, Wang M, Feng M, Yang F, Li L, Zhao J, Chang C, Dong H, Xie T, Chen J. Li Q, et al. Cancer Sci. 2021 May;112(5):1695-1706. doi: 10.1111/cas.14861. Epub 2021 Apr 6. Cancer Sci. 2021. PMID: 33605517 Free PMC article. Review.
Cited by
-
Liu D, Yu H, Pang Q, Zhang X. Liu D, et al. Front Physiol. 2018 Aug 14;9:1023. doi: 10.3389/fphys.2018.01023. eCollection 2018. Front Physiol. 2018. PMID: 30154726 Free PMC article.
-
Ramms DJ, Raimondi F, Arang N, Herberg FW, Taylor SS, Gutkind JS. Ramms DJ, et al. Pharmacol Rev. 2021 Oct;73(4):155-197. doi: 10.1124/pharmrev.120.000269. Pharmacol Rev. 2021. PMID: 34663687 Free PMC article. Review.
-
Zhao F, Zhou H, Li S, An Q, Fang Q, Luo Y, Hickford JGH. Zhao F, et al. Animals (Basel). 2021 Sep 14;11(9):2690. doi: 10.3390/ani11092690. Animals (Basel). 2021. PMID: 34573656 Free PMC article.
-
Roles of A-Kinase Anchoring Proteins and Phosphodiesterases in the Cardiovascular System.
Ercu M, Klussmann E. Ercu M, et al. J Cardiovasc Dev Dis. 2018 Feb 20;5(1):14. doi: 10.3390/jcdd5010014. J Cardiovasc Dev Dis. 2018. PMID: 29461511 Free PMC article. Review.
-
Ko HJ, Chiou SJ, Wong YH, Wang YH, Lai Y, Chou CH, Wang C, Loh JK, Lieu AS, Cheng JT, Lin YT, Lu PJ, Fann MJ, Huang CF, Hong YR. Ko HJ, et al. J Clin Med. 2019 Oct 21;8(10):1751. doi: 10.3390/jcm8101751. J Clin Med. 2019. PMID: 31640277 Free PMC article.
References
-
- Skroblin P., Grossmann S., Schäfer G., Rosenthal W., and Klussmann E. (2010) Mechanisms of protein kinase a anchoring. Int. Rev. Cell Mol. Biol. 283, 235–330 - PubMed
-
- McSorley T., Stefan E., Henn V., Wiesner B., Baillie G. S., Houslay M. D., Rosenthal W., and Klussmann E. (2006) Spatial organisation of AKAP18 and PDE4 isoforms in renal collecting duct principal cells. Eur. J. Cell Biol. 85, 673–678 - PubMed
MeSH terms
Substances
LinkOut - more resources
Full Text Sources
Other Literature Sources
Molecular Biology Databases
Miscellaneous