The axolotl limb blastema: cellular and molecular mechanisms driving blastema formation and limb regeneration in tetrapods - PubMed
- ️Thu Jan 01 2015
Review
. 2015 May 11;2(2):54-71.
doi: 10.1002/reg2.32. eCollection 2015 Apr.
Affiliations
- PMID: 27499868
- PMCID: PMC4895312
- DOI: 10.1002/reg2.32
Review
The axolotl limb blastema: cellular and molecular mechanisms driving blastema formation and limb regeneration in tetrapods
Catherine McCusker et al. Regeneration (Oxf). 2015.
Abstract
The axolotl is one of the few tetrapods that are capable of regenerating complicated biological structures, such as complete limbs, throughout adulthood. Upon injury the axolotl generates a population of regeneration-competent limb progenitor cells known as the blastema, which will grow, establish pattern, and differentiate into the missing limb structures. In this review we focus on the crucial early events that occur during wound healing, the neural-epithelial interactions that drive the formation of the early blastema, and how these mechanisms differ from those of other species that have restricted regenerative potential, such as humans. We also discuss how the presence of cells from the different axes of the limb is required for the continued growth and establishment of pattern in the blastema as described in the polar coordinate model, and how this positional information is reprogrammed in blastema cells during regeneration. Multiple cell types from the mature limb stump contribute to the blastema at different stages of regeneration, and we discuss the contribution of these types to the regenerate with reference to whether they are "pattern-forming" or "pattern-following" cells. Lastly, we explain how an engineering approach will help resolve unanswered questions in limb regeneration, with the goal of translating these concepts to developing better human regenerative therapies.
Keywords: Ambystoma mexicanum; blastema; limb; positional information; regeneration.
Figures
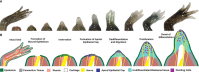
Axolotl limb blastema development. (A) Live images of the time course of limb blastema development showing an intact limb (left) and 1 day, 7, 9, 11, 13, 15, 17, 21, 25, and 31 days post amputation (consecutively to the right). (B) The key steps in the regenerative process are highlighted during blastema development. The tissue components are indicated by the color key. The intact limb is composed of multiple tissues including epidermis, connective tissue, cartilage, muscle, and nerves, which are organized in a specific way to generate functional structures (left). Within hours following an amputation, a wound epithelium covers the severed edge of the limb. Within days, this wound epithelium becomes innervated, and becomes a specialized signaling center known as the apical epithelial cap (AEC). The AEC induces dedifferentiation in the underlying stump tissue and attracts cells, which accumulate below the AEC. It is likely that positional interactions occur in the “dedifferentiation and migration” stage, because if insufficient positional disparity is present the blastema will continue to grow for a limited time but will not form limb structures. At later stages of development, the cells in the basal region of the blastema (closest to the stump) begin to differentiate, while the cells at the apical tip of the blastema remain in a proliferative and undifferentiated state (right). Over time, the blastema cells progressively differentiate into limb tissues from the basal to the apical regions of the blastema.
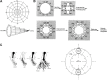
The polar coordinate model of regeneration. (A) The polar coordinate model for regeneration is based on the idea that cells in the limb field know their position relative to the circumferential axes of the limb (i.e., anterior/posterior and dorsal/ventral or coordinates 1−12) (top), and along the proximal−distal limb axis (A–E) (lower). (B) When the limb is amputated proximally (level “A”), a complete circle of positional values is present at the circumference of the wound. After the wound heals, cells from the different locations on the limb interact with cells that they do not normally interact with, stimulating a growth response that generates new cells with the intermediate (along the “shortest‐route”) circumferential positional values that correspond to the next proximal/distal level. The processes of intercalation and distalization are reiterated until all of the positional discontinuities are resolved and the pattern of the limb is completed. (C) If a “complete circle” of positional information is present in a regeneration‐competent environment, then a limb structure will form. This is exemplified by the formation of supernumerary limbs when the stylopod from a left forelimb is rotated 180° and grafted to the stylopod of the right forelimb, which generates positional discontinuities that result in the intercalation of complete circles of positional values at the dorsal/ventral poles (French et al. 1976; Bryant et al. 1981).
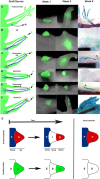
The stabilization of positional information in the blastema is controlled by nerve signaling. (A)−(F) Illustrations describing the origin of the grafted cells (left), live images of the grafted cells 1 and 3 weeks post‐grafting (middle), and whole mount skeleton staining (right) are provided to summarize the ectopic growth response when different blastema tissues are grafted into an accessory limb model (ALM). (A) Ectopic limbs are generated in the ALM when posterior skin is grafted into an anteriorly located wound site with a deviated nerve. (B) Grafts of early blastemas (EBs) do not induce the formation of ectopic limbs when grafted into an ALM; however, grafting an EB from a limb 3 days after it was denervated results in the formation of a segmented but incomplete regenerate (C). (D) Grafts of the apical tip of the late blastema (LB) do not induce the formation of ectopic limbs. (E) Similar to denervated EBs, denervated apical‐LBs induce the formation of segmented but incomplete regenerates. (F) Grafts of the basal region of the LB result in the formation of limb regenerates with completely patterned anterior/posterior and dorsal/ventral structures. (G) Our current model is that the positional information in the EB and the apical LB is labile or plastic, while the positional information has been stabilized in the stump and basal LB. If signaling from the nerves is removed, the positional information in the labile/plastic blastema cells prematurely stabilizes before the complete pattern of the missing structures has been intercalated, resulting in an incomplete regenerate (see C and E) (McCusker & Gardiner 2013). P, proximal; D, distal; I, intermediate; S, stable; L, labile.
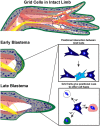
The pattern‐forming grid cells guide the behavior of pattern‐following cells. The cells that retain positional memory (dark blue) are located in the connective tissues that line all of the structures in the intact limb. When the limb is amputated, a regeneration‐competent environment is generated through nerve−epithelial interactions, which generate the apical epithelial cap (AEC) that dedifferentiates and recruits the grid cells from the tissues in different locations on the limb to accumulate below the AEC and interact (early blastema). The grid cells with differencing positional information (e.g., 4 and 6) induce an intercalary response to generate cells with the missing positional information (i.e., 5). At later stages of development (late blastema), the basal region of the blastema begins differentiating, and the grid cells provide positional cues to guide the behavior of other pattern‐following cell types (e.g., muscle, epithelial, and Schwann cells) that do not retain positional memory. At the same time, positional interactions continue to occur in the apical tip of the blastema to generate the pattern of the more distal structures in the regenerate.
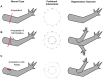
Amputation‐induced and supernumerary blastemas. (A) Amputation‐induced blastemas have a complete circle of positional information, which results in the formation of a complete limb. (B) 180° rotation of the limb results in the formation of blastema tissue with two complete circles of positional information, and thus results in the generation of two supernumerary limbs. (C) Grafting posterior skin into an anterior wound site results in the formation of a blastema with a complete circle of positional information, forming a single supernumerary limb.
Similar articles
-
Ghosh S, Roy S, Séguin C, Bryant SV, Gardiner DM. Ghosh S, et al. Dev Growth Differ. 2008 May;50(4):289-97. doi: 10.1111/j.1440-169X.2008.01000.x. Epub 2008 Mar 10. Dev Growth Differ. 2008. PMID: 18336582
-
Vieira WA, Wells KM, Raymond MJ, De Souza L, Garcia E, McCusker CD. Vieira WA, et al. Dev Biol. 2019 Jul 15;451(2):146-157. doi: 10.1016/j.ydbio.2019.04.008. Epub 2019 Apr 23. Dev Biol. 2019. PMID: 31026439 Free PMC article.
-
Neural control of growth and size in the axolotl limb regenerate.
Wells KM, Kelley K, Baumel M, Vieira WA, McCusker CD. Wells KM, et al. Elife. 2021 Nov 15;10:e68584. doi: 10.7554/eLife.68584. Elife. 2021. PMID: 34779399 Free PMC article.
-
Developmental aspects of spinal cord and limb regeneration.
Chernoff EAG, Stocum DL. Chernoff EAG, et al. Dev Growth Differ. 1995 Apr;37(2):133-147. doi: 10.1046/j.1440-169X.1995.t01-1-00002.x. Dev Growth Differ. 1995. PMID: 37281907 Review.
-
Avila-Martinez N, Gansevoort M, Verbakel J, Jayaprakash H, Araujo IM, Vitorino M, Tiscornia G, van Kuppevelt TH, Daamen WF. Avila-Martinez N, et al. Biomater Sci. 2023 Sep 12;11(18):6060-6081. doi: 10.1039/d3bm00835e. Biomater Sci. 2023. PMID: 37525590 Review.
Cited by
-
Mathavarajah S, Thompson AW, Stoyek MR, Quinn TA, Roy S, Braasch I, Dellaire G. Mathavarajah S, et al. J Exp Zool B Mol Dev Evol. 2024 May;342(3):241-251. doi: 10.1002/jez.b.23227. Epub 2023 Oct 25. J Exp Zool B Mol Dev Evol. 2024. PMID: 37877156
-
Appendage Regeneration in Vertebrates: What Makes This Possible?
Daponte V, Tylzanowski P, Forlino A. Daponte V, et al. Cells. 2021 Jan 27;10(2):242. doi: 10.3390/cells10020242. Cells. 2021. PMID: 33513779 Free PMC article. Review.
-
Tyler SEB. Tyler SEB. Front Physiol. 2017 Sep 4;8:627. doi: 10.3389/fphys.2017.00627. eCollection 2017. Front Physiol. 2017. PMID: 28928669 Free PMC article. Review.
-
Optogenetic manipulation of cellular communication using engineered myosin motors.
Zhang Z, Denans N, Liu Y, Zhulyn O, Rosenblatt HD, Wernig M, Barna M. Zhang Z, et al. Nat Cell Biol. 2021 Feb;23(2):198-208. doi: 10.1038/s41556-020-00625-2. Epub 2021 Feb 1. Nat Cell Biol. 2021. PMID: 33526902 Free PMC article.
-
Altın H, Delice B, Yıldırım B, Demircan T, Yıldırım S. Altın H, et al. Wound Repair Regen. 2024 Nov-Dec;32(6):826-839. doi: 10.1111/wrr.13207. Epub 2024 Aug 6. Wound Repair Regen. 2024. PMID: 39105277 Free PMC article.
References
-
- Albert, P. , Boilly, B. , Courty, J. & Barritault, D. (1987). Stimulation in cell culture of mesenchymal cells of newt limb blastemas by EDGF I or II (basic or acidic FGF). Cell Differentiation, 21, 63–68. - PubMed
-
- Bely, A.E. & Nyberg, K.G. (2010). Evolution of animal regeneration: re‐emergence of a field. Trends Ecol. Evol., 25, 161–170. - PubMed
-
- Brockes, J.P. (1997). Amphibian limb regeneration: rebuilding a complex structure. Science, 276, 81–87. - PubMed
-
- Bryant, S.V. & Bellairs, A.D. (1967). Tail regeneration in the lizards Anguis fragilis and Lacerta dugesii. Journal of the Linnean Society of London, Zoology, 46, 297–305.
Publication types
LinkOut - more resources
Full Text Sources
Other Literature Sources