Amplification of RNA by an RNA polymerase ribozyme - PubMed
- ️Fri Jan 01 2016
Amplification of RNA by an RNA polymerase ribozyme
David P Horning et al. Proc Natl Acad Sci U S A. 2016.
Abstract
In all extant life, genetic information is stored in nucleic acids that are replicated by polymerase proteins. In the hypothesized RNA world, before the evolution of genetically encoded proteins, ancestral organisms contained RNA genes that were replicated by an RNA polymerase ribozyme. In an effort toward reconstructing RNA-based life in the laboratory, in vitro evolution was used to improve dramatically the activity and generality of an RNA polymerase ribozyme by selecting variants that can synthesize functional RNA molecules from an RNA template. The improved polymerase ribozyme is able to synthesize a variety of complex structured RNAs, including aptamers, ribozymes, and, in low yield, even tRNA. Furthermore, the polymerase can replicate nucleic acids, amplifying short RNA templates by more than 10,000-fold in an RNA-catalyzed form of the PCR. Thus, the two prerequisites of Darwinian life-the replication of genetic information and its conversion into functional molecules-can now be accomplished with RNA in the complete absence of proteins.
Keywords: PCR; RNA enzyme; in vitro evolution; ribozyme; self-replication.
Conflict of interest statement
R.R.B. and G.F.J. appeared as coauthors of a paper published as Breaker RR, Joyce GF (2014) The expanding view of RNA and DNA function. Chem Biol 21(9):1059–1065. This paper was a Perspective commemorating the 20th anniversary of the journal.
Figures
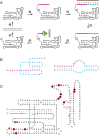
In vitro evolution of RNA polymerase ribozymes. (A) Selective amplification of ribozymes that extend a tethered RNA primer (magenta) on a separate RNA template (brown) to complete a 3′-truncated aptamer. (1) Attachment of the primer to the ribozyme via a photocleavable linker; (2) hybridization of the primer to the template; (3) extension of the primer by polymerization of NTPs (cyan); (4) capture of full-length materials by binding the aptamer portion to its immobilized ligand (green); (5) photocleavage to release the ribozyme portion; (6) reverse transcription and PCR amplification of the released ribozyme; and (7) transcription to generate progeny ribozymes. (B) Sequence and secondary structure of RNA aptamers that bind either cyanocobalamin (Left) or GTP (Right). (C) Sequence and secondary structure of the 24-3 polymerase. Red circles indicate mutations relative to WT, with color intensity indicating mutations that were universal (dark), common (medium), or sporadic (light) among the clones that were sequenced.
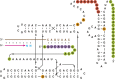
Sequence and secondary structure of the WT polymerase ribozyme. Changes relative to the original class I polymerase (12) are highlighted, with the 5′-terminal tag that binds to a complementary region of the template (14) shown in purple, a set of activity-enhancing mutations derived from the tC19Z ribozyme (14) shown in orange, and the site of a large deletion within the 3′-terminal domain (13) shown in red. Nucleotides that were added to enable primer binding for mutagenic PCR are shown in green.
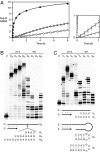
Improved activity of the 24-3 polymerase. (A) Primer extension on an 11-nt C-rich template catalyzed by the 24-3 (black), WT (gray), or tC19Z (white) polymerase. Data represent the average of three replicates, with SDs in the range of 0.03–0.09 nt/min and not visible in the figure. Initial rates based on a linear fit of the data (at right, with replicates shown) were 1.2, 0.012, and 0.005 nt/min, respectively. Reaction conditions: 1 μM polymerase, 0.4 μM primer, 0.5 μM template, 4 mM each NTP, 200 mM MgCl2, and 50 mM Tris (pH 8.3) at 17 °C. (B) Primer extension on templates containing a 20-nt C-rich sequence interrupted by a purine-rich sequence of 0, 4, 8, or 12 nt (R0, R4, R8, or R12, respectively). (C) Primer extension on templates containing stem-loops of either 4 (S4) or 6 bp (S6), in comparison with the 5′ portion of the stem alone (Y4 or Y6, respectively). Reaction conditions for B and C as above, but using 100 nM polymerase, 40 nM primer, and 50 nM template and incubating for 24 h. P, unextended primer. Dots indicate full-length extension products, with extralength material corresponding to continued extension through the spacer and portion of the template that is complementary to the sequence tag of the ribozyme.
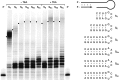
Primer extension by the 24-3 polymerase on templates containing stem-loop structures. The templates contained a 4-nt (S4), 6-nt (S6), or 8-nt (S8,8a–c) stem closed by a stable tetraloop (either 5′-UCCG-3′ or 5′-GCAA-3′). Reaction conditions: either 50 nM polymerase, 40 nM primer, 50 nM template, and no tetraethylammonium chloride (TEA); or 400 nM polymerase, 160 nM primer, 200 nM template, and 1 M TEA; both with 4 mM each NTP, 200 mM MgCl2, and 50 mM Tris (pH 8.3) at 17 °C for 72 h. P, unextended primer. Dots indicate full-length extension products, with extralength material corresponding to continued extension through the spacer and portion of the template that is complementary to the sequence tag of the ribozyme.
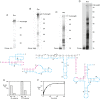
Synthesis of functional RNAs by the 24-3 polymerase. Synthesis of (A) the cyanocobalamin aptamer after 24 h, (B) the GTP aptamer after 24 h, (C) the F1 ligase ribozyme after 24 h, and (D) yeast phenylalanyl-tRNA after 72 h. Polymerization conditions as in Fig. 2B, but using 50 nM polymerase. Dots indicate full-length extension products. The slightly reduced mobility of full-length aptamers generated by the polymerase compared with those from chemical synthesis is due to a small difference (29 Da) in the composition of the 5′-fluorescein label. Sequence and secondary structure of the primer (magenta) and polymerized portion (cyan) of each RNA are shown below the respective gel images; substrates for the F1 ligase are shown in black. (E) Fraction of cyanocobalamin (B12) or GTP aptamers bound and eluted from corresponding ligand-derived resins, comparing synthetic (Syn) and ribozyme-synthesized (Rz) aptamers and a nonbinding control RNA (–). (F) Catalytic activity of the full-length, ribozyme-synthesized F1 ligase in a reaction mixture containing 1 μM ligase, 0.2 μM 5′ substrate, 10 μM 3′ substrate, 25 mM MgCl2, and 50 mM EPPS (pH 8.5) at 20 °C. Data were fit to the following equation: yield = yieldmax (1 – e–kobs•t), where yieldmax = 13% and kobs = 0.11 min–1.
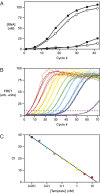
RNA-catalyzed exponential amplification of RNA (riboPCR). (A) Amplification of a 24-nt template, tracking either the template (white circles) or complement (black circles) compared with the reaction with no input template (white and black squares, respectively). Reaction conditions: 0.4 µM polymerase, 0.2 µM each primer, ±1 nM starting template, 4 mM each NTP, 50 mM MgCl2, 0.9 M TPA, 6% (wt/vol) PEG8000, and 50 mM Tris (pH 8.3), with thermal cycles of 72 °C for 2 s and then 17 °C for 4 h. (B) Real-time riboPCR of a 20-nt template, monitored by FRET between two fluorescently labeled primers. Reaction conditions as above, but with starting template concentrations of 10 nM (dark red), 3.2 nM (red), 1 nM (orange), 0.32 nM (yellow), 0.1 nM (light green), 32 pM (green), 10 pM (cyan), 3.2 pM (blue), 1 pM (indigo), or 0 (violet) and with thermal cycles of 68 °C for 1 s and then 17 °C for 30 min. Dashed line indicates a threshold FRET value of 10% of maximum. For each starting template concentration, three replicates were obtained (thin lines) and averaged (thick line) to determine a cycle-to-threshold (Ct) value. (C) Semilog plot of Ct vs. starting template concentration, demonstrating a linear relationship with slope of –8.8, corresponding to per-cycle amplification of 1.3-fold.
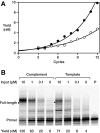
RiboPCR amplification of a 20-nt template. (A) Yield of newly synthesized template (white circles) and complement (black circles) over the first 12 cycles, starting with 200 pM template. Data were fit to a single exponential growth equation, giving growth rates of 0.27 and 0.18 per cycle for the complement and template, respectively, which corresponds to 1.2- to 1.3-fold amplification per cycle. (B) Amplification starting from low initial template concentrations to yield both complement and template. Reaction conditions: 0.4 µM polymerase, 0.2 µM each primer, 0, 0.1, 1, or 10 pM starting template, 4 mM each NTP, 50 mM MgCl2, 0.81 M tetrapropylammonium chloride, 6% (wt/vol) PEG8000, and 50 mM Tris (pH 8.3), with thermal cycles of 68 °C for 3 s and then 17 °C for 30 min.
Similar articles
-
An RNA polymerase ribozyme that synthesizes its own ancestor.
Tjhung KF, Shokhirev MN, Horning DP, Joyce GF. Tjhung KF, et al. Proc Natl Acad Sci U S A. 2020 Feb 11;117(6):2906-2913. doi: 10.1073/pnas.1914282117. Epub 2020 Jan 27. Proc Natl Acad Sci U S A. 2020. PMID: 31988127 Free PMC article.
-
Ribozyme-catalyzed transcription of an active ribozyme.
Wochner A, Attwater J, Coulson A, Holliger P. Wochner A, et al. Science. 2011 Apr 8;332(6026):209-12. doi: 10.1126/science.1200752. Science. 2011. PMID: 21474753
-
Amplification of an RNA ligase ribozyme under alternating temperature conditions.
Kim KS, Oh S, Yea SS, Yoon MY, Kim DE. Kim KS, et al. FEBS Lett. 2008 Aug 6;582(18):2745-52. doi: 10.1016/j.febslet.2008.07.013. Epub 2008 Jul 14. FEBS Lett. 2008. PMID: 18625231
-
Evolutionary origins and directed evolution of RNA.
Ellington AD, Chen X, Robertson M, Syrett A. Ellington AD, et al. Int J Biochem Cell Biol. 2009 Feb;41(2):254-65. doi: 10.1016/j.biocel.2008.08.015. Epub 2008 Aug 19. Int J Biochem Cell Biol. 2009. PMID: 18775793 Review.
-
In search of an RNA replicase ribozyme.
McGinness KE, Joyce GF. McGinness KE, et al. Chem Biol. 2003 Jan;10(1):5-14. doi: 10.1016/s1074-5521(03)00003-6. Chem Biol. 2003. PMID: 12573693 Review.
Cited by
-
The Story of RNA Folding, as Told in Epochs.
Herschlag D, Bonilla S, Bisaria N. Herschlag D, et al. Cold Spring Harb Perspect Biol. 2018 Oct 1;10(10):a032433. doi: 10.1101/cshperspect.a032433. Cold Spring Harb Perspect Biol. 2018. PMID: 30275276 Free PMC article. Review.
-
Walton T, DasGupta S, Duzdevich D, Oh SS, Szostak JW. Walton T, et al. Proc Natl Acad Sci U S A. 2020 Mar 17;117(11):5741-5748. doi: 10.1073/pnas.1914367117. Epub 2020 Mar 2. Proc Natl Acad Sci U S A. 2020. PMID: 32123094 Free PMC article.
-
Rolling circle RNA synthesis catalyzed by RNA.
Kristoffersen EL, Burman M, Noy A, Holliger P. Kristoffersen EL, et al. Elife. 2022 Feb 2;11:e75186. doi: 10.7554/eLife.75186. Elife. 2022. PMID: 35108196 Free PMC article.
-
Systematic minimization of RNA ligase ribozyme through large-scale design-synthesis-sequence cycles.
Nomura Y, Yokobayashi Y. Nomura Y, et al. Nucleic Acids Res. 2019 Sep 26;47(17):8950-8960. doi: 10.1093/nar/gkz729. Nucleic Acids Res. 2019. PMID: 31504757 Free PMC article.
-
An RNA polymerase ribozyme that synthesizes its own ancestor.
Tjhung KF, Shokhirev MN, Horning DP, Joyce GF. Tjhung KF, et al. Proc Natl Acad Sci U S A. 2020 Feb 11;117(6):2906-2913. doi: 10.1073/pnas.1914282117. Epub 2020 Jan 27. Proc Natl Acad Sci U S A. 2020. PMID: 31988127 Free PMC article.
References
-
- Gilbert W. The RNA world. Nature. 1986;319(6055):618.
-
- Joyce GF. The antiquity of RNA-based evolution. Nature. 2002;418(6894):214–221. - PubMed
-
- Attwater J, Holliger P. A synthetic approach to abiogenesis. Nat Methods. 2014;11(5):495–498. - PubMed
-
- Pressman A, Blanco C, Chen IA. The RNA world as a model system to study the origin of life. Curr Biol. 2015;25(19):R953–R963. - PubMed
Publication types
MeSH terms
Substances
LinkOut - more resources
Full Text Sources
Other Literature Sources