Covalent Modifiers: A Chemical Perspective on the Reactivity of α,β-Unsaturated Carbonyls with Thiols via Hetero-Michael Addition Reactions - PubMed
- ️Sun Jan 01 2017
Covalent Modifiers: A Chemical Perspective on the Reactivity of α,β-Unsaturated Carbonyls with Thiols via Hetero-Michael Addition Reactions
Paul A Jackson et al. J Med Chem. 2017.
Abstract
Although Michael acceptors display a potent and broad spectrum of bioactivity, they have largely been ignored in drug discovery because of their presumed indiscriminate reactivity. As such, a dearth of information exists relevant to the thiol reactivity of natural products and their analogues possessing this moiety. In the midst of recently approved acrylamide-containing drugs, it is clear that a good understanding of the hetero-Michael addition reaction and the relative reactivities of biological thiols with Michael acceptors under physiological conditions is needed for the design and use of these compounds as biological tools and potential therapeutics. This Perspective provides information that will contribute to this understanding, such as kinetics of thiol addition reactions, bioactivities, as well as steric and electronic factors that influence the electrophilicity and reversibility of Michael acceptors. This Perspective is focused on α,β-unsaturated carbonyls given their preponderance in bioactive natural products.
Figures
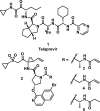
HCV protease inhibitors.
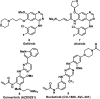
Reversible and irreversible EGFR inhibitors.
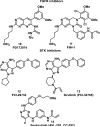
FGFR and Btk inhibitors.
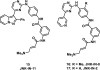
Pan JNK 1/2/3 inhibitors.
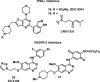
PI3Kα and VEGFR-2 inhibitors.
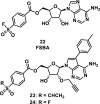
Inhibitors of Src kinases.
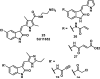
Nek2 inhibitors.
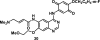
Dual EGFR/VEGFR-2 inhibitor.
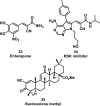
Rapidly reversible nitrile-containing Michael acceptors.
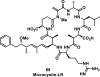
Example of a microcystin.
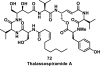
Structure of thalassospiramide A.
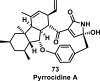
Structure of pyrrocidine A.
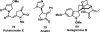
Natural products containing α-methylene, α-benzylidene, or α-ethylidene lactams.
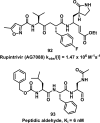
Inhibitors of human rhinovirus 3C protease (HRV-3CP).
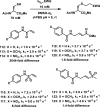
Michael acceptors and pseudo-first order reaction rates with N-acetyl-cysteine methyl ester.
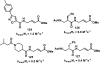
Irreversible papain inhibitors.
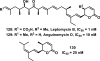
Inhibitors of CRM1-mediated nucleocytoplasmic transport and their IC50 values.
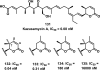
Kozusamycin A with analogs and cytotoxicity towards HPAC cells.
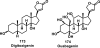
Examples of cardenolide natural products containing a butenolide.
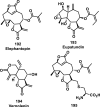
Structures of α-methylene-γ-lactone-containing natural products.
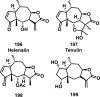
Structures of pseudoguaianolides.
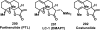
Natural product α-methylene-γ-lactones and prodrug derivative (fumarate salt of 201 not shown for clarity).

Structures of arglabin and derivatives.
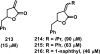
Reduction in cytotoxicity (IC50) when substituents are on the exocyclic methylene.
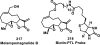
Melampomagnolide B and biotinylated derivative used for pulldown studies.
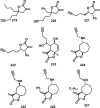
Library of α-methylene-γ-lactones containing terminal alkynes used as biological probes for the discovery of novel anti-microbial targets.
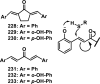
Styryl dienones and proposed mechanism for ortho-hydroxy substituent effect.
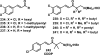
Styryl ketones and similar Mannich bases.
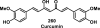
Structure of curcumin.
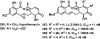
Resorcylic acid lactones with IC50 values for inhibition of TNFα-PLAP.
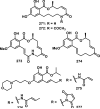
Synthetic resorcylic acid lactone analogs.
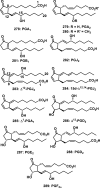
Selected CyPGs structures.
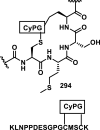
Proposed structure of CyPG crosslinking H-Ras C-terminal peptide (K170-K185).
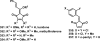
Natural product (351-354) and synthetic (355-357) cyclopentenediones with biological activity.
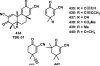
α-Nitrile cyclohexenone dually activated Michael acceptors.
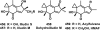
Illudin natural products and synthetic derivatives.
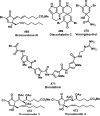
Examples of Bioactive α-haloacrolyl compounds.
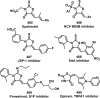
Examples of biologically active rhodanines.
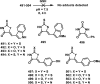
Rhodanines and analogs that did not form detectable adducts with GSH.
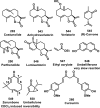
Compounds reactive toward cysteamine.
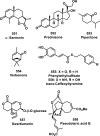
Compounds nonreactive toward cysteamine.
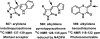
Classification of scaffolds by 13C NMR chemical shift values.
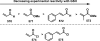
Relative experimental rates of GSH addition to α,β-unsaturated carbonyls.
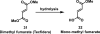
Dimethyl Fumarate Hydrolysis
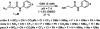
Relative Rates of GSH Addition to N-Arylacrylamides
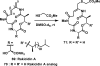
Rakicidin A and Analog that Forms a 1,6-Addition Product with Methyl Thioglycolate
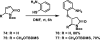
Thiol Addition to Pyrrolinones
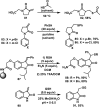
Thiol Adduct Formation with α-Methylene-γ-lactams and Oxindoles
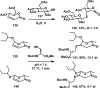
Thiol Addition to Unsaturated Sugars
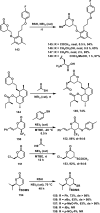
Thiol Addition to α,β-Unsaturated-δ-valerolactones
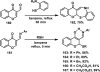
Thiol Addition to Dually Activated Chromones
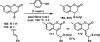
Thiol Addition Reactions to Coumarins
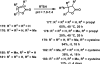
Effect of Alkyl Substitution on Thiol Addition to Butenolides
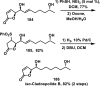
Thiol Adducts as Double Bond Protecting Groups
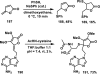
Thiol Addition to γ-Methylene or γ-Alkylidene Butenolides
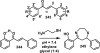
Generic Chalcone and Addition of Cysteamine to 2' Hydroxy Chalcone
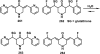
Reversibility of Glutathione Adducts of Curcumin Analogs
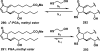
Equilibrium Formation of Thiol Adducts of PGA1 and Δ7-PGA1 Methyl Esters
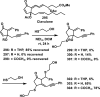
Formation of Thiol Adducts with Clavulone Derivatives
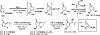
Reactions and Reversibility of Clavulone Derivatives with Thiols
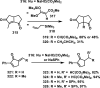
Nucleophilic Addition to Exocyclic vs Endocyclic Enones of Cyclopentenones
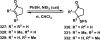
Equilibrium Formation of Thiol Adducts with Cyclopentenones
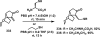
Cysteine and Propanethiol Addition to a Triquinane
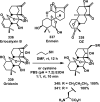
Selected Kaurane Natural Products and the Reaction of Oridonin with Thiols
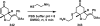
Addition of Cysteamine to a Cryptocaryone Analog
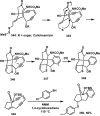
Mechanism of Thiol Activation of Calicheamicin and Thiol Addition to a Derivative
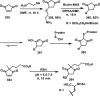
Synthesis of Sulfenic Acid Probe and Thiol Reactivity of Cyclopentenediones
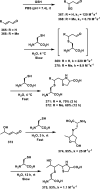
Reactions of Aldehydes with Thiols
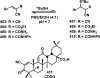
Dually Activated Michael Acceptors
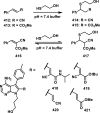
Reversibility of Dually Activated Michael Acceptors and RSK2 Inhibitors
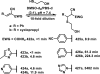
Tunable Reversibility of α-Heteroaromatic-Substituted Acrylonitriles
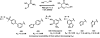
Effect of β-Substituent on the Reversibility of Thiol Addition to Dually Activated Michael Acceptors
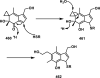
Mechanism for Direct Thiol Alkylation of Hydroxymethylacylfulvene
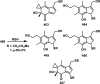
Products Obtained from the Reaction of HMAF with Thiols
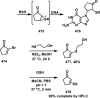
Reactions of α-Bromocyclopentenone with Thiols and DNA
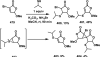
Products of Thiol Addition to α-Halo Butenolide with Proposed Intermediate
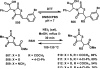
Reversible Additions of Thiols to Rhodanines and Related Scaffolds
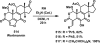
Reaction of Thiol and Amine Nucleophiles with Wortmannin
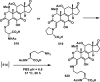
Crossover Experiments Showing the Reversibility of Wortmannin Adducts
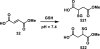
Reaction of Monomethyl Fumarate with GSH
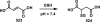
Reaction of Fumaric Acid with GSH
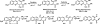
pH Dependence of Thiol Reactive Quinolines
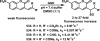
Coumarin Based Fluorogenic Probes and Second Order Rates Constants for GSH Addition
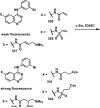
Fluorescence of Quinazoline Michael Acceptors upon Covalent Modification of a Cysteine in c-Src
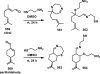
Reaction of α,β-Unsaturated Aldehydes with Cysteamine
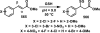
Methyl Cinnamates in Order of Decreasing Rates of GSH Addition
Similar articles
-
Krenske EH, Petter RC, Houk KN. Krenske EH, et al. J Org Chem. 2016 Dec 2;81(23):11726-11733. doi: 10.1021/acs.joc.6b02188. Epub 2016 Nov 8. J Org Chem. 2016. PMID: 27934455
-
Paasche A, Schiller M, Schirmeister T, Engels B. Paasche A, et al. ChemMedChem. 2010 Jun 7;5(6):869-80. doi: 10.1002/cmdc.201000020. ChemMedChem. 2010. PMID: 20401893 Free PMC article.
-
Dynamic thiol exchange with β-sulfido-α,β-unsaturated carbonyl compounds and dithianes.
Joshi G, Anslyn EV. Joshi G, et al. Org Lett. 2012 Sep 21;14(18):4714-7. doi: 10.1021/ol301781u. Epub 2012 Aug 30. Org Lett. 2012. PMID: 22934665 Free PMC article.
-
Thiol-addition reactions and their applications in thiol recognition.
Yin C, Huo F, Zhang J, Martínez-Máñez R, Yang Y, Lv H, Li S. Yin C, et al. Chem Soc Rev. 2013 Jul 21;42(14):6032-59. doi: 10.1039/c3cs60055f. Chem Soc Rev. 2013. PMID: 23703585 Review.
-
Piesche M, Roos J, Kühn B, Fettel J, Hellmuth N, Brat C, Maucher IV, Awad O, Matrone C, Comerma Steffensen SG, Manolikakes G, Heinicke U, Zacharowski KD, Steinhilber D, Maier TJ. Piesche M, et al. Front Pharmacol. 2020 Sep 3;11:1297. doi: 10.3389/fphar.2020.01297. eCollection 2020. Front Pharmacol. 2020. PMID: 33013366 Free PMC article. Review.
Cited by
-
Li P, Tian X, Zhang D, Ou H, Huang Q, Jin W, Liu R. Li P, et al. BMC Pharmacol Toxicol. 2024 Jun 28;25(1):36. doi: 10.1186/s40360-024-00758-2. BMC Pharmacol Toxicol. 2024. PMID: 38943212 Free PMC article.
-
Chaudhuri S, Rogers DM, Hayes CJ, Inzani K, Hirst JD. Chaudhuri S, et al. J Chem Inf Model. 2024 Oct 14;64(19):7687-7697. doi: 10.1021/acs.jcim.4c01379. Epub 2024 Sep 12. J Chem Inf Model. 2024. PMID: 39265068 Free PMC article.
-
Thia-Michael Reaction: The Route to Promising Covalent Adaptable Networks.
Berne D, Ladmiral V, Leclerc E, Caillol S. Berne D, et al. Polymers (Basel). 2022 Oct 21;14(20):4457. doi: 10.3390/polym14204457. Polymers (Basel). 2022. PMID: 36298037 Free PMC article. Review.
-
Murakami Y, Kawata A, Suzuki S, Fujisawa S. Murakami Y, et al. In Vivo. 2018 Nov-Dec;32(6):1309-1322. doi: 10.21873/invivo.11381. In Vivo. 2018. PMID: 30348683 Free PMC article.
-
Cheng XR, Yu BT, Song J, Ma JH, Chen YY, Zhang CX, Tu PH, Muskat MN, Zhu ZG. Cheng XR, et al. Antioxidants (Basel). 2022 Dec 5;11(12):2406. doi: 10.3390/antiox11122406. Antioxidants (Basel). 2022. PMID: 36552614 Free PMC article.
References
-
- Schöwobel JAH, Koleva YK, Enoch SJ, Bajot F, Hewitt M, Madden JC, Roberts DW, Schultz TW, Cronin MTD. Measurement and Estimation of Electrophilic Reactivity for Predictive Toxicology. Chem. Rev. 2011;111:2562–2596. - PubMed
-
- Singh J, Petter RC, Baillie TA, Whitty A. The Resurgence of Covalent Drugs. Nat. Rev. Drug Disc. 2011;10:307–317. - PubMed
-
- Parsons ZD, Gates KS. Redox Regulation of Protein Tyrosine Phosphatases: Methods for Kinetic Analysis of Covalent Enzyme Inactivation. In: Cadenas E, Packer L, editors. Methods in Enzymology Volume 528: Hydrogen Peroxide and Cell Signaling Part C. Vol. 528. Elsevier Science Publishing Co Inc; San Diego: 2013. pp. 130–154. - PubMed
- Kitz R, Wilson IB. Esters of Methanesulfonic Acid as Irreversible Inhibitors of Acetylcholinesterase. J. Biol. Chem. 1962;237:3245–3249. - PubMed
- Nagahara N, Sawada N, Nakagawa T. Affinity Labeling of a Catalytic Site, Cysteine 247, in Rat Mercaptopyruvate Sulfurtransfera se by Chloropyruvate as an Analog of a Substrate. Biochimie. 2004;86:723–729. - PubMed
MeSH terms
Substances
LinkOut - more resources
Full Text Sources
Other Literature Sources
Chemical Information
Research Materials