Rules for Shaping Neural Connections in the Developing Brain - PubMed
- ️Sun Jan 01 2017
Review
Rules for Shaping Neural Connections in the Developing Brain
Elena Kutsarova et al. Front Neural Circuits. 2017.
Abstract
It is well established that spontaneous activity in the developing mammalian brain plays a fundamental role in setting up the precise connectivity found in mature sensory circuits. Experiments that produce abnormal activity or that systematically alter neural firing patterns during periods of circuit development strongly suggest that the specific patterns and the degree of correlation in firing may contribute in an instructive manner to circuit refinement. In fish and amphibians, unlike amniotic vertebrates, sensory input directly drives patterned activity during the period of initial projection outgrowth and innervation. Experiments combining sensory stimulation with live imaging, which can be performed non-invasively in these simple vertebrate models, have provided important insights into the mechanisms by which neurons read out and respond to activity patterns. This article reviews the classic and recent literature on spontaneous and evoked activity-dependent circuit refinement in sensory systems and formalizes a set of mechanistic rules for the transformation of patterned activity into accurate neuronal connectivity in the developing brain.
Keywords: Hebbian plasticity; activity-dependent; colliculus; optic tectum; retinal ganglion cell; retinotectal; review; visual system.
Figures
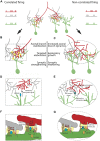
Cellular and molecular mechanisms underlying the instructive role of patterned neuronal activity in retinotectal map refinement. A retinal ganglion cell (RGC) axon of interest (red) synapses onto tectal neuron dendrites (green) in (A) The red axon (left branch) is co-active with its neighboring RGCs (gray) and its firing pattern is therefore correlated with the firing pattern of the tectal neuron on which it synapses. The right branch of the red axon is not co-active with its neighbors and its firing pattern is not correlated with the firing pattern of its synaptic partner. Synapses are depicted as yellow circles. Synaptic strength is represented by the size of the yellow circle. (B) Correlated firing patterns of an RGC with its partnering tectal neuron instruct an increase in synaptic strength and stabilization of the axonal arbor allowing for local targeted arbor elaboration as new branches tend to form at existing synapses. (C) Non-correlated firing of an RGC with its neighboring axons and its postsynaptic partner instructs synaptic weakening and an increase in axonal branch dynamics, accompanied by exploratory growth in search of a better partner. The effects of patterned neuronal activity on structural remodeling and synaptic efficacy are schematized in (D–G), respectively. (D) Shows a zoom-in of the area of the box in (B) depicting a “stabilizing retrograde signal” downstream of activation of N-methyl D-aspartate receptor (NMDAR) which encodes axonal branch stabilization and targeted elaboration. (E) Zoom-in of the ellipse in (C) Plausible mechanisms instructing axonal exploratory growth and branch destabilization due to the lack of correlated firing with the neighboring RGC inputs and the postsynaptic partner include: 1. Axo-axonal signal, released by the firing neighboring inputs (gray); 2.“Exploratory retrograde signal”, unmasked by the inactivation of NMDAR; 3. Cell-autonomous activity-dependent signal, released by the red neuron or acting intracellularly. (F) Zoom-in depicting molecular mechanisms underlying synaptic strengthening and stabilization of the red axon from (B). Correlated firing of the RGC axon (red) with its neighboring axons (gray) and the tectal neuron (green) results in both release of glutamate containing vesicles (dark red) and postsynaptic depolarization. This satisfies the conditions required for release of the Mg2+ block from the channel pore of the NMDAR (blue), allowing for cation influx. Ca2+ triggers a molecular cascade resulting in insertion of more AMPAR (orange) in the membrane. A retrograde signal downstream of NMDAR encoding higher probability of vesicle fusion in the red axon (depicted as higher number of vesicles in dark red). Increase in synaptic efficacy is accompanied by strong homophilic (brown) and heterophilic interactions of adhesion molecules (light blue and pink). The strength of the interaction is represented by the number of pairs of adhesion molecules. (G) Zoom-in showing the change is synaptic efficacy in (C). Non-correlated firing of the red axon with its neighbors and its partnering tectal neuron prevents opening of the channel pore of NMDAR, leading to AMPAR endocytosis, lower probability of glutamate release and weaker interaction of adhesive molecules.
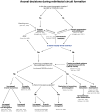
Flow-chart of decisions RGCs face during retinotectal map formation and refinement.
Similar articles
-
Penn AA, Shatz CJ. Penn AA, et al. Pediatr Res. 1999 Apr;45(4 Pt 1):447-58. doi: 10.1203/00006450-199904010-00001. Pediatr Res. 1999. PMID: 10203134 Review.
-
Pietri T, Romano SA, Pérez-Schuster V, Boulanger-Weill J, Candat V, Sumbre G. Pietri T, et al. Cell Rep. 2017 May 2;19(5):939-948. doi: 10.1016/j.celrep.2017.04.015. Cell Rep. 2017. PMID: 28467907 Free PMC article.
-
An instructive role for patterned spontaneous retinal activity in mouse visual map development.
Xu HP, Furman M, Mineur YS, Chen H, King SL, Zenisek D, Zhou ZJ, Butts DA, Tian N, Picciotto MR, Crair MC. Xu HP, et al. Neuron. 2011 Jun 23;70(6):1115-27. doi: 10.1016/j.neuron.2011.04.028. Neuron. 2011. PMID: 21689598 Free PMC article.
-
Enhanced visual activity in vivo forms nascent synapses in the developing retinotectal projection.
Aizenman CD, Cline HT. Aizenman CD, et al. J Neurophysiol. 2007 Apr;97(4):2949-57. doi: 10.1152/jn.00452.2006. Epub 2007 Jan 31. J Neurophysiol. 2007. PMID: 17267761
-
Retinal waves: implications for synaptic learning rules during development.
Butts DA. Butts DA. Neuroscientist. 2002 Jun;8(3):243-53. doi: 10.1177/1073858402008003010. Neuroscientist. 2002. PMID: 12061504 Review.
Cited by
-
Altered synaptic and firing properties of cerebellar Purkinje cells in a mouse model of ARSACS.
Ady V, Toscano-Márquez B, Nath M, Chang PK, Hui J, Cook A, Charron F, Larivière R, Brais B, McKinney RA, Watt AJ. Ady V, et al. J Physiol. 2018 Sep;596(17):4253-4267. doi: 10.1113/JP275902. Epub 2018 Jul 19. J Physiol. 2018. PMID: 29928778 Free PMC article.
-
Stentian structural plasticity in the developing visual system.
Rahman TN, Munz M, Kutsarova E, Bilash OM, Ruthazer ES. Rahman TN, et al. Proc Natl Acad Sci U S A. 2020 May 19;117(20):10636-10638. doi: 10.1073/pnas.2001107117. Epub 2020 May 4. Proc Natl Acad Sci U S A. 2020. PMID: 32366647 Free PMC article.
-
Spontaneous Afferent Activity Carves Olfactory Circuits.
Redolfi N, Lodovichi C. Redolfi N, et al. Front Cell Neurosci. 2021 Mar 9;15:637536. doi: 10.3389/fncel.2021.637536. eCollection 2021. Front Cell Neurosci. 2021. PMID: 33767612 Free PMC article.
-
To Stick or Not to Stick: The Multiple Roles of Cell Adhesion Molecules in Neural Circuit Assembly.
Moreland T, Poulain FE. Moreland T, et al. Front Neurosci. 2022 Apr 28;16:889155. doi: 10.3389/fnins.2022.889155. eCollection 2022. Front Neurosci. 2022. PMID: 35573298 Free PMC article. Review.
-
A transgenic zebrafish model for in vivo long-term imaging of retinotectal synaptogenesis.
Du XF, Xu B, Zhang Y, Chen MJ, Du JL. Du XF, et al. Sci Rep. 2018 Sep 19;8(1):14077. doi: 10.1038/s41598-018-32409-y. Sci Rep. 2018. PMID: 30232367 Free PMC article.
References
Publication types
MeSH terms
LinkOut - more resources
Full Text Sources
Other Literature Sources