Chirality in microbial biofilms is mediated by close interactions between the cell surface and the substratum - PubMed
Chirality in microbial biofilms is mediated by close interactions between the cell surface and the substratum
Liselotte Jauffred et al. ISME J. 2017 Jul.
Abstract
From microbial biofilms to human migrations, spatial competition is central to the evolutionary history of many species. The boundary between expanding populations is the focal point of competition for space and resources and is of particular interest in ecology. For all Escherichia coli strains studied here, these boundaries move in a counterclockwise direction even when the competing strains have the same fitness. We find that chiral growth of bacterial colonies is strongly suppressed by the expression of extracellular features such as adhesive structures and pili. Experiments with other microbial species show that chiral growth is found in other bacteria and exclude cell wall biosynthesis and anisotropic shape as the primary causes of chirality. Instead, intimate contact with the substratum is necessary for chirality. Our results demonstrate that through a handful of surface molecules cells can fundamentally reorganize their migration patterns, which might affect intra- and interspecific competitions through colony morphology or other mechanisms.
Conflict of interest statement
The authors declare no conflict of interest.
Figures
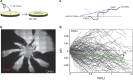
Competition experiments between neutral E. coli strains expressing different colors, either yellow fluorescent protein (YFP) (yellow) or cyan fluorescent protein (CFP) (cyan). (a) Sketch of the experiment where the two species are mixed 1:1, diluted 1/10, and inoculated on an agar plate where the borders between competing expanding strains are visible. (b) An example of an expanding E. coli DH5α colony is shown from the agar side; the cells transformed with CFP appear white and those with YFP black in this image. The radius of the light-blue dashed circle equals r0. The scale bar corresponds to 1 mm. (c) Illustration of the parameterization, (r, φ(r)), of a point on the sector boundary where r is the length of the radial vector (blue) from the center of the colony and φ(r) is the angle (denoted with orange) between the direction of the sector boundary (dashed orange line) and the radial vector. (d) Parametrization, φ(r), of individual E. coli DH5α sector boundaries (N=146) plotted on semilog scales. The green dots show the average boundary, 〈φ(r)〉, and the full green line shows the best linear fit to the average boundary. As clear from Equation (1), the slope of the fit is the dimensionless measure of chirality: v⊥/v∥=tan(θ). Note that, although the behavior of individual boundaries appear erratic due to random wandering of the boundaries, the average over 146 colonies shows a clear negative slope due to chirality. The values of v⊥/v∥ and θ for all strains investigated are given in Table 2.
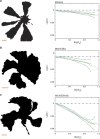
The sector boundaries show different degrees of chirality depending on expression of surface structures. The left panels show examples of intensity thresholded images (displayed from the agar side) of different E. coli MG1655 mutants in competition experiments; the scale bars are 1 mm. The right panels show the average boundary angle, 〈φ(r)〉 (green dotted line), a linear fit (full green line) and one standard error of the mean (blue punctuated line). (a) Results from competition experiments with the mother strain, MG1655; this colony expansion is only slightly chiral (N=369). (b) Competition experiments with the MG1655fim strain lacking pili (N=831). This strain exhibits more chiral borders than MG1655. (c) Competition experiments with the double deleted MG1655fimflu strain lacking both Antigen 43 and pili (N=636). This double deletion causes even more pronounced chirality compared with the single deletion mutants.
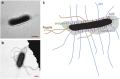
Electron microscopy images as well as a sketch of the E. coli and the surface structures investigated. (a, b) Transmission electron microscopy images of MG1655. The scale bar is 1 μm. (c) A sketch of E. coli with its extracellular constituents: Antigen 43, pili, curli fimbriae, colanic acid and flagella are explicitly drawn (not to scale). The body of the bacteria is shown in black.
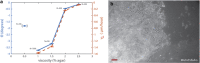
Effect of agar on chirality of sector boundaries and a microscopy image of the sector–sector and colony–agar boundaries. (a) Chirality measured θ (blue points and full line) and the border velocity, v⊥ (red points and punctuated line) are plotted for different agar concentrations; here MG1655fimflu E. coli strain with high chirality was used. The number of boundaries analyzed, N, are noted for each experiment and the error bars signify one s.e.m. At 0.5 % agar, the colony growth was severely compromised compared with other experiments. To indicate this, we put the corresponding θ value in parenthesis. (b) Fluorescent microscopy image (the YFP channel) of sector–sector and colony–agar boundaries in the MG1655fimflu strain. The scale bar is 10 μm.
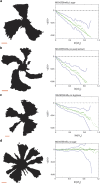
Effect of substratum substitution. The left panels show examples of intensity thresholded images (displayed from the agar side) of the E. coli MG1655fimflu mutant in competition experiments on LB medium with systematic replacements of constituents; the scale bars are 1 mm. The right panels show the average boundary angle, 〈φ(r)〉 (green dotted line), a linear fit (full green line) and one s.e.m. (blue punctuated line). (a) Results from control competition experiments on normal LB medium (1.2% agar) (N=222). (b) Replacement of yeast extract by 1 μg ml−1 B1, 1 m
mMgCl2, and 10 m
msodium phosphate (N=223). (c) Replacement of tryptone and NaCl by 1% decolorized casamino acids (N=337). (d) Replacement of agar by 1.2% agarose (N=410).
Similar articles
-
Flower-like patterns in multi-species bacterial colonies.
Xiong L, Cao Y, Cooper R, Rappel WJ, Hasty J, Tsimring L. Xiong L, et al. Elife. 2020 Jan 14;9:e48885. doi: 10.7554/eLife.48885. Elife. 2020. PMID: 31933477 Free PMC article.
-
Uhlich GA, Rogers DP, Mosier DA. Uhlich GA, et al. Foodborne Pathog Dis. 2010 Aug;7(8):935-43. doi: 10.1089/fpd.2009.0503. Foodborne Pathog Dis. 2010. PMID: 20367070
-
Yoshihara A, Nobuhira N, Narahara H, Toyoda S, Tokumoto H, Konishi Y, Nomura T. Yoshihara A, et al. Colloids Surf B Biointerfaces. 2015 Jul 1;131:67-72. doi: 10.1016/j.colsurfb.2015.04.038. Epub 2015 Apr 23. Colloids Surf B Biointerfaces. 2015. PMID: 25956746
-
Role of bacterial cell surface structures in Escherichia coli biofilm formation.
Van Houdt R, Michiels CW. Van Houdt R, et al. Res Microbiol. 2005 Jun-Jul;156(5-6):626-33. doi: 10.1016/j.resmic.2005.02.005. Epub 2005 Mar 22. Res Microbiol. 2005. PMID: 15950122 Review.
-
Thinking about bacterial populations as multicellular organisms.
Shapiro JA. Shapiro JA. Annu Rev Microbiol. 1998;52:81-104. doi: 10.1146/annurev.micro.52.1.81. Annu Rev Microbiol. 1998. PMID: 9891794 Review.
Cited by
-
Chiral twisting in a bacterial cytoskeletal polymer affects filament size and orientation.
Shi H, Quint DA, Grason GM, Gopinathan A, Huang KC. Shi H, et al. Nat Commun. 2020 Mar 16;11(1):1408. doi: 10.1038/s41467-020-14752-9. Nat Commun. 2020. PMID: 32179732 Free PMC article.
-
Genetic mixing and demixing on expanding spherical frontiers.
García Vázquez A, Mitarai N, Jauffred L. García Vázquez A, et al. ISME Commun. 2024 Jan 22;4(1):ycae009. doi: 10.1093/ismeco/ycae009. eCollection 2024 Jan. ISME Commun. 2024. PMID: 38524760 Free PMC article.
-
Intra-colony channels in E. coli function as a nutrient uptake system.
Rooney LM, Amos WB, Hoskisson PA, McConnell G. Rooney LM, et al. ISME J. 2020 Oct;14(10):2461-2473. doi: 10.1038/s41396-020-0700-9. Epub 2020 Jun 17. ISME J. 2020. PMID: 32555430 Free PMC article.
-
Cell organelle-based analysis of cell chirality.
Fan J, Zhang H, Rahman T, Stanton DN, Wan LQ. Fan J, et al. Commun Integr Biol. 2019 Apr 24;12(1):78-81. doi: 10.1080/19420889.2019.1605277. eCollection 2019. Commun Integr Biol. 2019. PMID: 31143366 Free PMC article.
-
Fields C, Levin M. Fields C, et al. Commun Integr Biol. 2020 Feb 18;13(1):27-38. doi: 10.1080/19420889.2020.1729601. eCollection 2020. Commun Integr Biol. 2020. PMID: 32128026 Free PMC article.
References
-
- Ali A, Grosskinsky S. (2010). Pattern formation through genetic drift at expanding population fronts. Adv Complex Syst 13: 349–366.
-
- Bachmann BJ. (1996). Derivations and genotypes of some mutant derivatives of Escherichia coli K-12. In: Neidhardt FC editor, Escherichia Coli and Salmonella Typhimurium: Cellular and Molecular Biology. ASM Press: Washington, DC, pp 2460–2488.
-
- Ben-Jacob E, Cohen I, Levine H. (2000). Cooperative self-organization of microorganisms. Adv Phys 49: 395–554.
-
- Ben-Jacob E, Cohen I, Shochet O, Tenenbaum A. (1995). Cooperative formation of chiral patterns during growth of bacterial colonies. Phys Rev Lett 75: 2899–2902. - PubMed
MeSH terms
LinkOut - more resources
Full Text Sources
Other Literature Sources