Structural Basis for Guide RNA Processing and Seed-Dependent DNA Targeting by CRISPR-Cas12a - PubMed
- ️Sun Jan 01 2017
Structural Basis for Guide RNA Processing and Seed-Dependent DNA Targeting by CRISPR-Cas12a
Daan C Swarts et al. Mol Cell. 2017.
Abstract
The CRISPR-associated protein Cas12a (Cpf1), which has been repurposed for genome editing, possesses two distinct nuclease activities: endoribonuclease activity for processing its own guide RNAs and RNA-guided DNase activity for target DNA cleavage. To elucidate the molecular basis of both activities, we determined crystal structures of Francisella novicida Cas12a bound to guide RNA and in complex with an R-loop formed by a non-cleavable guide RNA precursor and a full-length target DNA. Corroborated by biochemical experiments, these structures reveal the mechanisms of guide RNA processing and pre-ordering of the seed sequence in the guide RNA that primes Cas12a for target DNA binding. Furthermore, the R-loop complex structure reveals the strand displacement mechanism that facilitates guide-target hybridization and suggests a mechanism for double-stranded DNA cleavage involving a single active site. Together, these insights advance our mechanistic understanding of Cas12a enzymes and may contribute to further development of genome editing technologies.
Keywords: CRISPR RNA; CRISPR-Cas; Cas12a; Cas9; Cpf1; R-loop; crRNA processing; nuclease; seed sequence; target DNA cleavage.
Copyright © 2017 Elsevier Inc. All rights reserved.
Figures
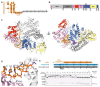
(A) Sequence of the crRNA guide (see also Table S1). Nucleotides that are ordered in the structure are colored orange, unordered nucleotides are colored grey. (B) Schematic diagram of the domain organization of FnCas12a. REC: Recognition. PI: Protospacer Adjacent Motif (PAM) interacting. WED: Wedge. BH: Bridge Helix. Nuc: Nuclease. Both the WED and RuvC domains are formed by three discontinuous segments of the protein sequence. For the genomic context and purification details of FnCas12a, see Figure S1. (C) Overall structure of the FnCas12a-crRNA complex. Domains are colored according to the scheme in panel B. For alignments of the structures of the binary complexes of FnCas12a and LbCas12a, see Figure S2. (D) Close-up view of the crRNA seed sequence. Hydrogen bonds between FnCas12a residues and the seed sequence are displayed as dashed lines. For details of FnCas12a-crRNA binding interactions, see Figure S3. For a sequence alignment of Cas12a orthologs in which the conserved residues involved in crRNA seed sequence pre-ordering are indicated, see Figure S4. (E) Target DNA complementarity to the crRNA seed sequence is critical for cleavage. Top: Schematic representation of the crRNA (orange) and target DNA sequences used in the nuclease activity assays (see also Table S1 and Table S2). Numbering indicates the position of the mismatches between the crRNA and DNA targets used in the nuclease activity assays. Bottom: FnCas12a-crRNA complexes were incubated with pre-linearized plasmid DNA substrates containing a wild type (WT) target sequence or sequences with mismatches in the target site. Cleavage products were resolved by agarose gel electrophoresis. LIN: linearized plasmid substrate. M: 1kb DNA Ladder. For EMSA binding assays demonstrating the importance of the crRNA seed sequence in target binding, see Figure S5.
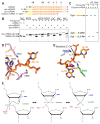
(A) Schematic representation of pre-crRNA substrates. Pre-crRNAX contains a deoxyuridine nucleotide at position (-19). Cy5-pre-crRNA is covalently labeled with a Cy5 fluorophore at the 5’ end of a truncated pre-crRNA sequence. (B) FnCas12a-mediated pre-crRNA processing depends on the presence of a ribose 2'-hydroxyl group in the nucleotide upstream of the scissile phosphate. FnCas12a was incubated with pre-cRNA1 or pre-crRNAX in the presence of chelating agents or divalent cations. Processing products were analyzed by denaturing polyacrylamide gel electrophoresis. (C) FnCas12a-mediated pre-crRNA processing generates a 5' product containing a 2',3'-cyclic phosphate. FnCas12a was incubated with Cy5-pre-crRNA, after which processing products were treated with Alkaline Phosphatase (AP; removes 3’-end phosphates) or T4 Polynucleotide Kinase (PNK; removes 2’,3’-cyclic phosphates). Reaction products were analyzed by denaturing polyacrylamide gel electrophoresis and fluorescence detection. (D) Close-up view of the pre-crRNA processing site in FnCas12a bound to pre-crRNAX. For a sequence alignment of Cas12a orthologs in which the conserved residues involved in pre-crRNA processing are indicated, see Figure S4. (E) Structural model of bona fide pre-crRNA substrate in which nucleotide U(-19) contains a (modeled) 2’-hydroxyl group, based on panel D. The 2’-hydroxyl group is positioned in close proximity of Lys869, suggesting that Lys869 acts as a general base in the catalytic mechanism. (F) Proposed model for the acid-base catalytic mechanism of pre-crRNA processing by FnCas12a.
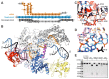
(A) Sequences of the crRNA guide and DNA target (see also Table S1). Structurally disordered nucleotides are colored grey. (B) Overall structure of the FnCas12a R-loop complex. The nucleic acid strands are colored as in panel A. For alignments of the structures of the ternary complexes of FnCas12a and AsCas12a, see Figure S2. For conformational rearrangements in FnCas12a upon transition from the binary to the ternary complex, see Figure S6. For details of FnCas12a-crRNA-DNA interactions, see Figure S7. (C) Close-up view of the interactions with the non-target DNA strand at the PAM-proximal end of the R-loop. (D) Close-up view of the interactions with the target DNA strand at the PAM-proximal end of the R-loop. (E) Mutations of the FnCas12a residues interacting with the displaced non-target DNA strand impair substrate DNA cleavage. Wild-type and mutated FnCas12a was incubated with crRNA and a pre-linearized plasmid DNA substrate. Cleavage products were analyzed by agarose gel electrophoresis. LIN: linearized plasmid substrate. M: 1kb DNA Ladder. ΔRLI: Ala-Gly3 substitution of R-loop interacting residues Thr698–Ser702. 1 and 2: products of linear DNA cleavage.
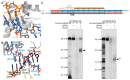
(A) Close-up view of the PAM-distal end of the crRNA-target DNA heteroduplex. Tyr410 stacking caps the crRNA-target DNA heteroduplex at base pair U20-dA(-20) and prevents duplex formation beyond nucleotide U20 of the crRNA. (B) Close-up view of the PAM-distal flank of the R-loop. The PAM-distal segments of the target and non-target DNA strands hybridize in the central cavity of FnCas12a, between the REC2 and Nuc domains. (C) Exonuclease III footprinting analysis of the FnCas12a–R-loop complex. Top: schematic representation of the crRNA sequence (orange) and target DNA sequences used in the footprinting assay (see also Table S1). FnCas12aDM-crRNA was bound to target DNA duplexes in which either the target strand (TS) or the non-target strand (NTS) was 5’-labeled with ATTO532. The resulting complexes were treated with Exonuclease III (ExoIII) and cleavage products were analyzed by denaturing polyacrylamide gel electrophoresis and fluorescence detection. M: Marker generated by S1 treatment of the labeled DNA strand. * and ** indicate the TS and NTS products formed by ExoIII when the dsDNA is protected by the FnCas12aDM-crRNA1 complex. Nucleotides removed from the TS and from the NTS by ExoIII are colored red in the schematic diagram at the top of the panel.
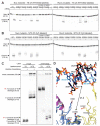
(A) Target strand DNA cleavage is inhibited by specific mutations in the RuvC domain (right panel), but not by any of the mutations in the Nuc domain (left panel). Wild-type and mutated FnCas12a were incubated with crRNA and a dsDNA oligonucleotide substrate in which the target strand was labeled with 3’-ATTO532. Cleavage products were analyzed by denaturing polyacrylamide gel electrophoresis and fluorescence detection. (B) Non-target strand DNA cleavage is completely inhibited by specific mutations in the RuvC domain (right panel), but not by any of the mutations in the Nuc domain (left panel). Wild-type and mutated FnCas12a were incubated with crRNA and a dsDNA oligonucleotide substrate in which the non-target strand was labeled with 5’-Cy5. Cleavage products were analyzed as in (A). (C) Cleavage of both target and non-target DNA strands generates products containing a 5’-phosphate and 3’-hydroxyl groups. dsDNA oligonucleotide substrates, labeled as indicated with covalently attached fluorophores, were cleaved with the FnCas12a-crRNA complex. Cleavage products were further incubated in the presence or absence of alkaline phosphatase and analyzed by denaturing polyacrylamide gel electrophoresis and fluorescence detection. (D) Close-up view of the RuvC and Nuc domain residues involved in DNA cleavage and the PAM-distal part of the R-loop. Arrow indicates the displacement of the scissile phosphate in the target DNA strand from the RuvC domain active site. For a modeled non-target strand in the RuvC catalytic site of FnCas12a, see Figure S8.
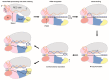
Cas12a catalyzes the processing of its own crRNA guide and pre-orders crRNA nucleotides 1-5 in the seed sequence. PAM recognition by a preformed binding site in Cas12a positions the target DNA in register with the pre-ordered crRNA seed sequence. Local strand separation in the vicinity of the PAM allows the target strand to base pair to the crRNA seed sequence to initiate R-loop formation. The R-loop is formed by directional guide-target hybridization. The RNA-DNA heteroduplex is terminated after 20 base pairs at the PAM-distal end. The resulting R-loop structure can adopt two alternative conformations that result in the insertion of either the target or the non-target DNA strand in a single catalytic site located at the interface of the RuvC and Nuc domains.
Similar articles
-
The CRISPR-associated DNA-cleaving enzyme Cpf1 also processes precursor CRISPR RNA.
Fonfara I, Richter H, Bratovič M, Le Rhun A, Charpentier E. Fonfara I, et al. Nature. 2016 Apr 28;532(7600):517-21. doi: 10.1038/nature17945. Epub 2016 Apr 20. Nature. 2016. PMID: 27096362
-
Mechanistic Insights into the cis- and trans-Acting DNase Activities of Cas12a.
Swarts DC, Jinek M. Swarts DC, et al. Mol Cell. 2019 Feb 7;73(3):589-600.e4. doi: 10.1016/j.molcel.2018.11.021. Epub 2019 Jan 10. Mol Cell. 2019. PMID: 30639240 Free PMC article.
-
Structural Basis for the Canonical and Non-canonical PAM Recognition by CRISPR-Cpf1.
Yamano T, Zetsche B, Ishitani R, Zhang F, Nishimasu H, Nureki O. Yamano T, et al. Mol Cell. 2017 Aug 17;67(4):633-645.e3. doi: 10.1016/j.molcel.2017.06.035. Epub 2017 Aug 3. Mol Cell. 2017. PMID: 28781234 Free PMC article.
-
The Revolution Continues: Newly Discovered Systems Expand the CRISPR-Cas Toolkit.
Murugan K, Babu K, Sundaresan R, Rajan R, Sashital DG. Murugan K, et al. Mol Cell. 2017 Oct 5;68(1):15-25. doi: 10.1016/j.molcel.2017.09.007. Mol Cell. 2017. PMID: 28985502 Free PMC article. Review.
-
Editor's cut: DNA cleavage by CRISPR RNA-guided nucleases Cas9 and Cas12a.
Swartjes T, Staals RHJ, van der Oost J. Swartjes T, et al. Biochem Soc Trans. 2020 Feb 28;48(1):207-219. doi: 10.1042/BST20190563. Biochem Soc Trans. 2020. PMID: 31872209 Free PMC article. Review.
Cited by
-
Jabalera Y, Tascón I, Samperio S, López-Alonso JP, Gonzalez-Lopez M, Aransay AM, Abascal-Palacios G, Beisel CL, Ubarretxena-Belandia I, Perez-Jimenez R. Jabalera Y, et al. Nat Biotechnol. 2024 Oct 31. doi: 10.1038/s41587-024-02461-3. Online ahead of print. Nat Biotechnol. 2024. PMID: 39482449
-
Structural basis for two metal-ion catalysis of DNA cleavage by Cas12i2.
Huang X, Sun W, Cheng Z, Chen M, Li X, Wang J, Sheng G, Gong W, Wang Y. Huang X, et al. Nat Commun. 2020 Oct 16;11(1):5241. doi: 10.1038/s41467-020-19072-6. Nat Commun. 2020. PMID: 33067443 Free PMC article.
-
Utilization of nicking properties of CRISPR-Cas12a effector for genome editing.
Kim CH, Lee WJ, Oh Y, Lee Y, Lee HK, Seong JB, Lim KS, Park SJ, Huh JW, Kim YH, Kim KM, Hur JK, Lee SH. Kim CH, et al. Sci Rep. 2024 Feb 9;14(1):3352. doi: 10.1038/s41598-024-53648-2. Sci Rep. 2024. PMID: 38336977 Free PMC article.
-
Mechanisms for target recognition and cleavage by the Cas12i RNA-guided endonuclease.
Zhang H, Li Z, Xiao R, Chang L. Zhang H, et al. Nat Struct Mol Biol. 2020 Nov;27(11):1069-1076. doi: 10.1038/s41594-020-0499-0. Epub 2020 Sep 7. Nat Struct Mol Biol. 2020. PMID: 32895556 Free PMC article.
-
The compact Casπ (Cas12l) 'bracelet' provides a unique structural platform for DNA manipulation.
Sun A, Li CP, Chen Z, Zhang S, Li DY, Yang Y, Li LQ, Zhao Y, Wang K, Li Z, Liu J, Liu S, Wang J, Liu JG. Sun A, et al. Cell Res. 2023 Mar;33(3):229-244. doi: 10.1038/s41422-022-00771-2. Epub 2023 Jan 17. Cell Res. 2023. PMID: 36650285 Free PMC article.
References
-
- Cho SW, Kim S, Kim JM, Kim J-S. Targeted genome engineering in human cells with the Cas9 RNA-guided endonuclease. Nat Biotechnol. 2013;31:230–232. - PubMed
MeSH terms
Substances
LinkOut - more resources
Full Text Sources
Other Literature Sources
Research Materials