Ion channel mechanisms underlying frequency-firing patterns of the avian nucleus magnocellularis: A computational model - PubMed
- ️Sun Jan 01 2017
Ion channel mechanisms underlying frequency-firing patterns of the avian nucleus magnocellularis: A computational model
Ting Lu et al. Channels (Austin). 2017.
Abstract
We have previously shown that late-developing avian nucleus magnocellularis (NM) neurons (embryonic [E] days 19-21) fire action potentials (APs) that resembles a band-pass filter in response to sinusoidal current injections of varying frequencies. NM neurons located in the mid- to high-frequency regions of the nucleus fire preferentially at 75 Hz, but only fire a single onset AP to frequency inputs greater than 200 Hz. Surprisingly, NM neurons do not fire APs to sinusoidal inputs less than 20 Hz regardless of the strength of the current injection. In the present study we evaluated intrinsic mechanisms that prevent AP generation to low frequency inputs. We constructed a computational model to simulate the frequency-firing patterns of NM neurons based on experimental data at both room and near physiologic temperatures. The results from our model confirm that the interaction among low- and high-voltage activated potassium channels (KLVA and KHVA, respectively) and voltage dependent sodium channels (NaV) give rise to the frequency-firing patterns observed in vitro. In particular, we evaluated the regulatory role of KLVA during low frequency sinusoidal stimulation. The model shows that, in response to low frequency stimuli, activation of large KLVA current counterbalances the slow-depolarizing current injection, likely permitting NaV closed-state inactivation and preventing the generation of APs. When the KLVA current density was reduced, the model neuron fired multiple APs per sinusoidal cycle, indicating that KLVA channels regulate low frequency AP firing of NM neurons. This intrinsic property of NM neurons may assist in optimizing response to different rates of synaptic inputs.
Keywords: NEURON; action potential; auditory brainstem; nucleus magnocellularis; voltage dependent potassium channel; voltage dependent sodium channel.
Figures
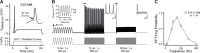
Mid- to high-frequency NM neurons show distinct firing patterns in response to sustained and sinusoidal current injections. (A) Representative voltage trace recorded from a late-developing NM neuron (E20) in response to sustained current injection shown below (325 pA, 100 ms). The strength of sustained current is 25% above threshold current (IThreshold = 260 pA). The inset marks the AP voltage threshold. In this and subsequent figures, schematic stimuli used to evoke responses are shown below representative traces. (B) Representative voltage traces in response to 5 (left, inset), 10 (left), 75 (middle), and 200 Hz (right) sinusoidal current injections recorded from the same NM neuron shown in (A). The strength of 10, 75, and 200 Hz sinusoidal current is 150% above threshold current (i.e., 650 pA*). In this and subsequent figures, * = 150% above threshold current. The arrow in the left panel points the maximum depolarizing value of the voltage response (i.e., -53 mV). In this and subsequent figures, brackets point the traces that are enlarged in the insets, insets shown with scale bar are due to space limitations, otherwise complete x and y axis are provided. Scale bar values for the insets are 25 mV/10 ms. (C) Population data (E19–21, n = 14) showing the firing probability per sinusoidal cycle, calculated as the number of APs divided by the total number of sinusoidal cycles, plotted as a function of stimulus frequency. Error bars = standard error of the mean. Data modified from Hong et al., 2016.
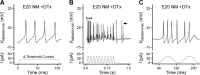
Application of DTx changes the firing patterns of NM neurons in response to sustained and sinusoidal current injections. (A) Representative voltage trace in response to sustained current injection (23 pA, 100 ms) during DTx (0.1 μM) application. Δ threshold current = reduction in current needed to elicit AP generation after DTx application. (B) Representative voltage trace in response to 10 Hz sinusoidal current injection (50 pA) during DTx application. The arrow marks spontaneous APs. The area indicated by the bracket is expanded and shown in (C). Traces shown in this figure were recorded from the same NM neuron shown in Fig. 1.
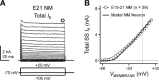
Current-voltage relationship of total potassium currents. (A) Representative KV current traces (Total IK) recorded from an NM neuron (E21) in response to membrane voltages clamped from −100 to +20 mV (voltage step = 5 mV, voltage duration = 100 ms). Circle at the end of current traces represent time window of measured steady-state KV currents (Total SS IK). (B) Total SS IK show a nonlinear change as a function of membrane voltages for NM neurons (empty symbols, mean ± standard error of the mean, E19–21, n = 39) and for the single-compartment model (solid line). Experimental data modified from Hong et al., 2016.
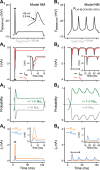
Simulation of NM neuron firing patterns to sustained current injection, before (A) and after (B) KLVA blockade. Results were obtained from single-compartment NM model. (A1–4) Responses induced by sustained current injections (325 pA, 100 ms). (B1–4) Responses induced by sustained current injections (50 pA, 100 ms) with 100% KLVA blockade. Traces shown are model output of membrane voltage (VMEMBRANE, A1 & B1), the NaV and KV currents (A2 & B2, INa and −IK,), NaV availability (h NaV) and probability of inactivation state (1-h NaV) (A3 & B3) and low- and high-voltage activated potassium currents (A4 & B4, KLVA and KHVA).
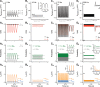
Simulation of NM neuron frequency-firing patterns to sinusoidal current injections. Results were obtained from single-compartment NM model. (A1–4 - D1–4) Responses induced by 5, 10, 75, and 200 Hz sinusoidal current injections, respectively. Traces shown are model output of membrane voltage (VMEMBRANE, A1-D1), the NaV and KV currents (A2-D2, INa and −IK,), NaV availability (h NaV) and probability of inactivation state (1-h NaV) (A3-D3) and low- and high-voltage activated potassium currents (A4-D4, KLVA and KHVA). Scale bar values are 25 mV/10 ms and 0.5 nA/10 ms for insets in the C1 & D1 and C2 & D2, respectively.
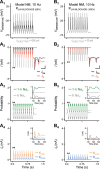
Simulation of the effect of KLVA blockade on model NM's firing in response to 10 Hz sinusoidal current injection. (A1–4 & B1–4) Responses induced by 10 Hz sinusoidal current injection with 55% and 100% KLVA blockade, respectively. Traces shown are model output of membrane voltage (VMEMBRANE, A1 & B1), the NaV and KV currents (A2 & B2, INa and −IK,), NaV availability (h NaV) and probability of inactivation state (1-h NaV) (A3 & B3) and low- and high-voltage activated potassium currents (A4 & B4, KLVA and KHVA). Scale bar values are 0.5 nA/20 ms for A2 & B2.
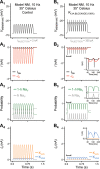
Simulation of the effect of temperature on model NM's firing in response to 10 Hz sinusoidal current injections under control (A) and with 100% KLVA blockade (B). Simulations were run at near physiologic temperature of 35°C. Traces shown are model output of membrane voltage (VMEMBRANE, A1 & B1), the NaV and KV currents (A2 & B2, INa and −IK,), NaV availability (h NaV) and probability of inactivation state (1-h NaV) (A3 & B3) and low- and high-voltage activated potassium currents (A4 & B4, KLVA and KHVA).
Comment in
-
Your genes decide what you are listening to.
Kopp-Scheinpflug C. Kopp-Scheinpflug C. Channels (Austin). 2017 Sep 3;11(5):355-356. doi: 10.1080/19336950.2017.1348870. Epub 2017 Jun 29. Channels (Austin). 2017. PMID: 28662361 Free PMC article. No abstract available.
Similar articles
-
Hong H, Wang X, Lu T, Zorio DAR, Wang Y, Sanchez JT. Hong H, et al. Front Cell Neurosci. 2018 Jun 26;12:175. doi: 10.3389/fncel.2018.00175. eCollection 2018. Front Cell Neurosci. 2018. PMID: 29997479 Free PMC article.
-
Resurgent sodium current promotes action potential firing in the avian auditory brainstem.
Hong H, Lu T, Wang X, Wang Y, Sanchez JT. Hong H, et al. J Physiol. 2018 Feb 1;596(3):423-443. doi: 10.1113/JP275083. Epub 2018 Jan 4. J Physiol. 2018. PMID: 29193076 Free PMC article.
-
Developmental Profile of Ion Channel Specializations in the Avian Nucleus Magnocellularis.
Hong H, Rollman L, Feinstein B, Sanchez JT. Hong H, et al. Front Cell Neurosci. 2016 Mar 30;10:80. doi: 10.3389/fncel.2016.00080. eCollection 2016. Front Cell Neurosci. 2016. PMID: 27065805 Free PMC article.
-
Hong H, Sanchez JT. Hong H, et al. J Exp Neurosci. 2018 Dec 12;12:1179069518815628. doi: 10.1177/1179069518815628. eCollection 2018. J Exp Neurosci. 2018. PMID: 30559595 Free PMC article. Review.
-
Mechanisms for the resonant property in rodent neurons.
Hashimoto K. Hashimoto K. Neurosci Res. 2020 Jul;156:5-13. doi: 10.1016/j.neures.2019.12.013. Epub 2019 Dec 20. Neurosci Res. 2020. PMID: 31870695 Review.
Cited by
-
The Roles of Potassium and Calcium Currents in the Bistable Firing Transition.
Borges FS, Protachevicz PR, Souza DLM, Bittencourt CF, Gabrick EC, Bentivoglio LE, Szezech JD Jr, Batista AM, Caldas IL, Dura-Bernal S, Pena RFO. Borges FS, et al. Brain Sci. 2023 Sep 20;13(9):1347. doi: 10.3390/brainsci13091347. Brain Sci. 2023. PMID: 37759949 Free PMC article.
-
Your genes decide what you are listening to.
Kopp-Scheinpflug C. Kopp-Scheinpflug C. Channels (Austin). 2017 Sep 3;11(5):355-356. doi: 10.1080/19336950.2017.1348870. Epub 2017 Jun 29. Channels (Austin). 2017. PMID: 28662361 Free PMC article. No abstract available.
-
Hong H, Wang X, Lu T, Zorio DAR, Wang Y, Sanchez JT. Hong H, et al. Front Cell Neurosci. 2018 Jun 26;12:175. doi: 10.3389/fncel.2018.00175. eCollection 2018. Front Cell Neurosci. 2018. PMID: 29997479 Free PMC article.
-
Resurgent sodium current promotes action potential firing in the avian auditory brainstem.
Hong H, Lu T, Wang X, Wang Y, Sanchez JT. Hong H, et al. J Physiol. 2018 Feb 1;596(3):423-443. doi: 10.1113/JP275083. Epub 2018 Jan 4. J Physiol. 2018. PMID: 29193076 Free PMC article.
-
The Role of Potassium and Calcium Currents in the Bistable Firing Transition.
Borges FS, Protachevicz PR, Souza DLM, Bittencourt CF, Gabrick EC, Bentivoglio LE, Szezech JD Jr, Batista AM, Caldas IL, Dura-Bernal S, Pena RFO. Borges FS, et al. bioRxiv [Preprint]. 2023 Aug 17:2023.08.16.553625. doi: 10.1101/2023.08.16.553625. bioRxiv. 2023. PMID: 37645875 Free PMC article. Updated. Preprint.
References
-
- Suta D, Popelar J, Syka J. Coding of communication calls in the subcortical and cortical structures of the auditory system. Physiol Res 2008; 57 Suppl 3:S149-159; PMID:18481905 - PubMed
-
- Velluti RA, Pedemonte M. In vivo approach to the cellular mechanisms for sensory processing in sleep and wakefulness. Cell Mol Neurobiol 2002; 22:501-16; PMID:12585677; https://doi.org/10.1023/A:1021956401616 - DOI - PubMed
-
- Wenstrup JJ, Nataraj K, Sanchez JT. Mechanisms of spectral and temporal integration in the mustached bat inferior colliculus. Frontiers Neural Circuits 2012; 6:75; PMID:23109917; https://doi.org/10.3389/fncir.2012.00075 - DOI - PMC - PubMed
-
- Carr CE, Soares D. Evolutionary convergence and shared computational principles in the auditory system. Brain Behavior Evolution 2002; 59:294-311; https://doi.org/10.1159/000063565 - DOI - PubMed
-
- Carr CE, Soares D, Parameshwaran S, Perney T. Evolution and development of time coding systems. Curr Opinion Neurobiol 2001; 11:727-33; PMID:11741025; https://doi.org/10.1016/S0959-4388(01)00276-8 - DOI - PubMed
MeSH terms
Substances
LinkOut - more resources
Full Text Sources
Other Literature Sources
Miscellaneous