Ion channel signaling influences cellular proliferation and phagocyte activity during axolotl tail regeneration - PubMed
Ion channel signaling influences cellular proliferation and phagocyte activity during axolotl tail regeneration
Brandon M Franklin et al. Mech Dev. 2017 Aug.
Abstract
Little is known about the potential for ion channels to regulate cellular behaviors during tissue regeneration. Here, we utilized an amphibian tail regeneration assay coupled with a chemical genetic screen to identify ion channel antagonists that altered critical cellular processes during regeneration. Inhibition of multiple ion channels either partially (anoctamin1/Tmem16a, anoctamin2/Tmem16b, KV2.1, KV2.2, L-type CaV channels and H/K ATPases) or completely (GlyR, GABAAR, KV1.5 and SERCA pumps) inhibited tail regeneration. Partial inhibition of tail regeneration by blocking the calcium activated chloride channels, anoctamin1&2, was associated with a reduction of cellular proliferation in tail muscle and mesenchymal regions. Inhibition of anoctamin 1/2 also altered the post-amputation transcriptional response of p44/42 MAPK signaling pathway genes, including decreased expression of erk1/erk2. We also found that complete inhibition via voltage gated K+ channel blockade was associated with diminished phagocyte recruitment to the amputation site. The identification of H+ pumps as required for axolotl tail regeneration supports findings in Xenopus and Planaria models, and more generally, the conservation of ion channels as regulators of tissue regeneration. This study provides a preliminary framework for an in-depth investigation of the mechanistic role of ion channels and their potential involvement in regulating cellular proliferation and other processes essential to wound healing, appendage regeneration, and tissue repair.
Copyright © 2017 Elsevier B.V. All rights reserved.
Figures
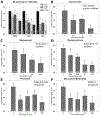
(A) All broad scale inhibitors of chloride channels (DNDS, DIDS and NFA) robustly reduced tail regeneration at concentrations of 100 nM and above. (B–D) Inhibitors of calcium activated chloride currents with CaCCinh-A01, T16a(inh)-A01 and Benzbromarone all reduced regenerative growth in a concentration dependent manner with maximal responses at 2, 100 and 0.33 μM respectively. (E & F) PTx and BCU were used to investigate the role of ligand-gated chloride channels and both exhibited robust inhibition of regeneration at all concentrations tested (* indicates p < 0.05 compare to control or concentration = 0; error bars are standard deviations, n values depicted on bar graphs represent biological replicates).
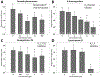
(A) While the broad scale potassium channel inhibitor TEA only reduced regenerative growth (B) the voltage-gated K+ channel blocker 4-AP exhibited concentration dependent inhibition. (C) KV2.1/2.2 channels that were inhibited with GTx-1E exhibited a slight reduction of regenerative growth at 1 and 5 μM but was lethal at all concentrations above 5 μM and (D) CB exhibited complete inhibition at 10 μM (* indicates p < 0.05 compare to control or concentration = 0; error bars are standard deviations, n values depicted on bar graphs represent biological replicates).
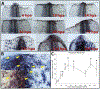
Phagocytes were stained in live regenerating axolotl embryos with neutral red at 6, 18, 24, 36, 48 (2 days), 72 (3 days), 120 (5 days), 144 (6 days) & 168 (7 days) hpa. Panel (A) depicts representative micrographs of each time point (amputation plane indicated by black dashed line) with enlarged photos in panels (B & B′) showing individual phagocytes. Representative (but not all) NR+ cells are indicated by yellow arrowheads. (C) Phagocytes were counted from 500 μM proximal of the amputation plane to the tip of the tail and normalized by tissue cross-sectional area. Phagocyte density steadily increased over the first few days following amputation peaking between days 2 and 3. This was followed by a trough in phagocyte density between days 3 and 5 that corresponded to increased tissue outgrowth, and then a second phagocyte density peak at day 6 (* indicates p < 0.05 compared to phagocyte density immediately following amputation; scale bars represent 1 mm; error bars are standard deviations). (For interpretation of the references to colour in this figure legend, the reader is referred to the web version of this article.)
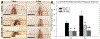
Phagocytes were stained in live animals with neutral red at 3 and 6 dpa. Panel (A) depicts representative micrographs of all time points and treatments (amputation plane indicated by black dashed line). (B) 4AP inhibits phagocyte activation and recruitment to the wound site at concentrations of either 5 or 25 μM. This response is concentration dependent at 6dpa but not 3dpa (* indicates p < 0.05 compared to control and ^ indicates p < 0.05 compared animals treated with 5 μM 4AP; scale bars represent 1 mm; error bars are standard deviations).
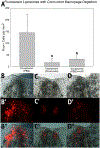
Phagocytes were labeled by IP injection of Fluorosome®-DiI 24 h prior to amputation and co-injected with either Encapsome® as a vehicle control (B-B″; n = 10), Clodrosome® for macrophage depletion (C-C″, n = 15) and a final group co-injected with Encapsome® and then incubated with 25 μM 4-AP following amputation for KV channel blockade (D-D”; n = 14). (* indicates p < 0.05 compared to control; error bars are standard deviations) Both macrophage depletion prior to amputation (C-C″) and KV channel blockade following amputation (D-D″) reduced the number of DiI+ cells at the wound site.
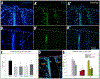
Compared to controls (n = 4) (A-A″), the proliferative response to amputation was reduced by broad scale chloride channel blockade with either DIDS (n = 4) or DNDS (n = 5) (C) as well as anoctamin-1 channel blockade with T16a(inh)-A01 (n = 5) (B-B″)) but not when animals were incubated in chloride free medium (n = 5) (C) (amputation plane indicated by white dashed line). Taken together these data suggest that chloride channel signaling is a critical step in the mechanism(s) driving proliferation in response to amputation. (* indicates p < 0.05 compared to control; error bars are standard deviations) (D&E) Neither DIDS nor T16a(inh)-A01 had an effect on proliferation in spinal cord (SC) or epidermal (ED) tissues. In mesenchymal tissues (MM) directly underlying the wound epidermis and within 500 μm of the amputation plane, proliferation was reduced from 46.6 ± 0.7% in control animals to either 20.0 ± 3.5% (DIDS) or 23.0 ± 2.9% (T16a(inh)-A01) in treated animals (* indicates p < 0.05 compared to control of same tissue type; error bars are standard deviations).
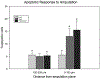
Apoptosis was assessed following amputation in 3 dpa animals and compared to apoptotic rates in tissue from un-amputated axolotl tails. Tissues were cut into transverse sections and then divided into groups consisting of sections of either from the tail tip to 100 μM distal of the amputation plane and a second group consisting of tissue from 150 to 250 μM distal of the amputation plane. No differenced were observed in the latter group but the former exhibited similar increases in apoptotic cells in both the control 3 dpa animals and 3 dpa animals treated with the Ano1 inhibitor (* indicates p < 0.05 compared to un-amputated tail tissue).
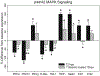
Quantitative Real-Time PCR was used to assess the mRNA expression of genes associated with the p44/42 MAPK (Erk 1/2) signaling pathway at 72 h post amputation (hpa) in regenerating axolotl tails and at the same time point in animals treated with 20 μM T16Ainh-A01. Expression in tail tissues from un-amputated animals was used as control/baseline. All genes other than PKCγ exhibit modulated expression at 3 dpa, which was attenuated by Ano1 blockade (* indicates p < 0.05 compared to control and ^ indicates p < 0.05 compared to normal 72 hpa animals; error bars are standard deviations).
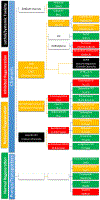
A comprehensive screen of ion channel blockers was used to identify specific channels involved in critical cellular processes of growth and regeneration such as proliferation, migration and differentiation. Drugs that did not affect regeneration are depicted in green, drugs that resulted in partial regeneration are depicted in yellow, drugs that completely inhibited regeneration (for any of the concentrations tested) are depicted in red and drugs that were lethal at all concentrations or caused systemic toxicity (identified by general atrophy, lethargy and/or tissue degeneration) are depicted in black. (For interpretation of the references to colour in this figure legend, the reader is referred to the web version of this article.)
Similar articles
-
Histone deacetylases are required for amphibian tail and limb regeneration but not development.
Taylor AJ, Beck CW. Taylor AJ, et al. Mech Dev. 2012 Sep-Dec;129(9-12):208-18. doi: 10.1016/j.mod.2012.08.001. Epub 2012 Aug 26. Mech Dev. 2012. PMID: 22947425
-
Ponomareva LV, Athippozhy A, Thorson JS, Voss SR. Ponomareva LV, et al. Comp Biochem Physiol C Toxicol Pharmacol. 2015 Dec;178:128-135. doi: 10.1016/j.cbpc.2015.06.004. Epub 2015 Jun 16. Comp Biochem Physiol C Toxicol Pharmacol. 2015. PMID: 26092703 Free PMC article.
-
Amputation-induced reactive oxygen species signaling is required for axolotl tail regeneration.
Al Haj Baddar NW, Chithrala A, Voss SR. Al Haj Baddar NW, et al. Dev Dyn. 2019 Feb;248(2):189-196. doi: 10.1002/dvdy.5. Epub 2018 Dec 21. Dev Dyn. 2019. PMID: 30569660 Free PMC article.
-
Exploring the role of microRNAs in axolotl regeneration.
Abo-Al-Ela HG, Burgos-Aceves MA. Abo-Al-Ela HG, et al. J Cell Physiol. 2021 Feb;236(2):839-850. doi: 10.1002/jcp.29920. Epub 2020 Jul 7. J Cell Physiol. 2021. PMID: 32638401 Review.
-
The physiological roles of anoctamin2/TMEM16B and anoctamin1/TMEM16A in chemical senses.
Dibattista M, Pifferi S, Hernandez-Clavijo A, Menini A. Dibattista M, et al. Cell Calcium. 2024 Jun;120:102889. doi: 10.1016/j.ceca.2024.102889. Epub 2024 Apr 18. Cell Calcium. 2024. PMID: 38677213 Review.
Cited by
-
Post-amputation reactive oxygen species production is necessary for axolotls limb regeneration.
Carbonell-M B, Zapata Cardona J, Delgado JP. Carbonell-M B, et al. Front Cell Dev Biol. 2022 Aug 26;10:921520. doi: 10.3389/fcell.2022.921520. eCollection 2022. Front Cell Dev Biol. 2022. PMID: 36092695 Free PMC article.
-
Salamander-like tail regeneration in the West African lungfish.
Verissimo KM, Perez LN, Dragalzew AC, Senevirathne G, Darnet S, Barroso Mendes WR, Ariel Dos Santos Neves C, Monteiro Dos Santos E, Nazare de Sousa Moraes C, Elewa A, Shubin N, Fröbisch NB, de Freitas Sousa J, Schneider I. Verissimo KM, et al. Proc Biol Sci. 2020 Sep 30;287(1935):20192939. doi: 10.1098/rspb.2019.2939. Epub 2020 Sep 16. Proc Biol Sci. 2020. PMID: 32933441 Free PMC article.
-
Dooling KE, Kim RT, Kim EM, Chen E, Abouelela A, Tajer BJ, Lopez NJ, Paoli JC, Powell CJ, Luong AG, Wu SYC, Thornton KN, Singer HD, Savage AM, Bateman J, DiTommaso T, Payzin-Dogru D, Whited JL. Dooling KE, et al. bioRxiv [Preprint]. 2024 Aug 31:2024.08.29.610385. doi: 10.1101/2024.08.29.610385. bioRxiv. 2024. PMID: 39257748 Free PMC article. Preprint.
-
MAPK/ERK Pathway as a Central Regulator in Vertebrate Organ Regeneration.
Wen X, Jiao L, Tan H. Wen X, et al. Int J Mol Sci. 2022 Jan 27;23(3):1464. doi: 10.3390/ijms23031464. Int J Mol Sci. 2022. PMID: 35163418 Free PMC article. Review.
-
Biomedical applications of electrical stimulation.
Zhao S, Mehta AS, Zhao M. Zhao S, et al. Cell Mol Life Sci. 2020 Jul;77(14):2681-2699. doi: 10.1007/s00018-019-03446-1. Epub 2020 Jan 23. Cell Mol Life Sci. 2020. PMID: 31974658 Free PMC article. Review.
References
-
- Adams DS, Masi A, Levin M, 2007. H+ pump-dependent changes in membrane voltage are an early mechanism necessary and sufficient to induce Xenopus tail regeneration. Development 134 (7), 1323–1335. - PubMed
-
- Baddar NWAH, et al., 2015. Sal-site: research resources for the Mexican Axolotl In: Kumar A, Simon A (Eds.), Salamanders in Regeneration Research: Methods and Protocols. Springer New York, New York, NY, pp. 321–336. - PubMed
-
- Balestrini L, et al., 2017. The natural compound sanguinarine perturbs the regenerative capabilities of planarians. Int. J. Dev. Biol 61 (1–2), 43–52. - PubMed
Publication types
MeSH terms
Substances
LinkOut - more resources
Full Text Sources
Other Literature Sources
Miscellaneous