Young Genes are Highly Disordered as Predicted by the Preadaptation Hypothesis of De Novo Gene Birth - PubMed
Young Genes are Highly Disordered as Predicted by the Preadaptation Hypothesis of De Novo Gene Birth
Benjamin A Wilson et al. Nat Ecol Evol. 2017 Jun.
Abstract
The phenomenon of de novo gene birth from junk DNA is surprising, because random polypeptides are expected to be toxic. There are two conflicting views about how de novo gene birth is nevertheless possible: the continuum hypothesis invokes a gradual gene birth process, while the preadaptation hypothesis predicts that young genes will show extreme levels of gene-like traits. We show that intrinsic structural disorder conforms to the predictions of the preadaptation hypothesis and falsifies the continuum hypothesis, with all genes having higher levels than translated junk DNA, but young genes having the highest level of all. Results are robust to homology detection bias, to the non-independence of multiple members of the same gene family, and to the false positive annotation of protein-coding genes.
Figures
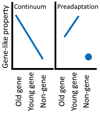
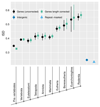
This result from the analysis of 15,347 mouse genes is unchanged by correction for evolutionary rate, and only becomes stronger after correction for length (green squares). Back-transformed central tendency estimates +/- one standard error come from a linear mixed model, where gene family, phylostratum, and length are random, fixed, and quantitative terms respectively. Importantly, this means that we do not treat genes as independent data points, but instead take into account phylogenetic confounding, and use gene families as independent data points. Length-corrected ISD values are with respect to a standardized length of 179 amino acids. Both young genes and old genes have higher ISD than intergenic sequences (blue diamond) and repeat-masked intergenic sequences (light blue triangle). Phylostrata on the x-axis are labeled according to the clade in which the oldest detectable homolog of a gene can be found. To minimize homology detection bias, the oldest phylostrata have been condensed into a single Pre-vertebrate phylostratum.
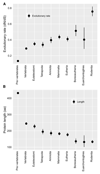
These properties are directly causal for homology detection bias, and hence there is no way to produce bias-corrected values as for Fig. 2. However, the statistical insignificance of rate-correction in Fig. 2 suggests that homology detection bias is negligible. Back-transformed central tendency estimates +/- one standard error come from a linear mixed model, where gene family and phylostratum are random and fixed terms respectively.
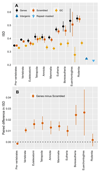
(A) ISD in real proteins (black circles) relative to amino acid scrambled controls (orange squares), and controls generated to have matched GC content (yellow diamonds), with error bars showing the back transform of the central tendency estimates +/- 1 standard error derived from mixed models as in Fig. 2. Excess ISD is driven primarily by amino acid composition, not GC content or precise amino acid order. (B) Paired comparisons show that the small excess in ISD relative to that predicted from amino acid composition is statistically significant (95% confidence intervals are shown) in all young genes except the very youngest, despite the broad confidence intervals in (A) that do not take into account the paired nature of the data.
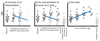
A) The continuum view posits the existence of “proto-genes” that have “characteristics intermediate between non-genic ORFs and genes”. Candidate proto-genes were classified on the basis of being annotated as ORFs, and having detectable sequence homology in sister species (without necessarily retention of approximate ORF boundaries), and Carvunis et al (2012) claimed to show a continuum of properties as a function of conservation level, shown as a greyscale. B) The same data can be explained without resorting to the existence of such intermediates. Sequence homology for ORFs that are not protein-coding genes (white circles) becomes more difficult to detect as a function of age, such that the proportion of true genes (black circles) increases with age, giving rise to the same observations as A. The downward trend in ISD arises as an example of Simpson’s paradox. C) By carefully excluding all non-genes, we see the true relationship between gene age and ISD, and compare it to intergenic control sequences that are definitely not protein-coding genes. Note that if true protein-coding genes were excluded in B (rather than excluding non-genes as in C), there would be no relationship with conservation levels.
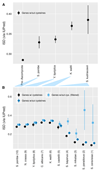
A) Back-transformed central tendency estimates +/- one standard error come from a linear mixed model, where gene family and phylostratum are random and fixed terms, respectively. Phylostrata are labeled according to the species most closely related to S. cerevisiae in which a homolog is still found, except for the “S. kudriavzevii” group, which includes younger genes found in at least two species. The analysis includes 5452 yeast genes that overlap with the genes used by Carvunis et al. (2012) with filtering indicated in Table 1. B) Using the age classifications of Carvunis et al. (2012) (Table 1, 2nd column), and ignoring gene family, we reproduce the trend of low ISD in young “proto-genes” using our slightly different ISD measurement. Standard means +/- one standard error are reported for untransformed ISD estimates. This trend is insensitive to whether cysteines are included (black circles) or excluded (blue diamonds) from the protein primary sequence. This trend disappears when we screen out “proto-genes” that lack strong evidence for a functional protein product (light-blue squares), by excluding genes whose age we could classify or which were unique to S. cerevisiae, and those classified as “dubious” in SGD (Table 1; last column). Correspondences between the ages assigned by the two phylostratigraphies are indicated with shaded triangles between the two figure parts.
Similar articles
-
Casola C. Casola C. Genome Biol Evol. 2018 Nov 1;10(11):2906-2918. doi: 10.1093/gbe/evy231. Genome Biol Evol. 2018. PMID: 30346517 Free PMC article.
-
A Continuum of Evolving De Novo Genes Drives Protein-Coding Novelty in Drosophila.
Heames B, Schmitz J, Bornberg-Bauer E. Heames B, et al. J Mol Evol. 2020 May;88(4):382-398. doi: 10.1007/s00239-020-09939-z. Epub 2020 Apr 7. J Mol Evol. 2020. PMID: 32253450 Free PMC article.
-
Readthrough Errors Purge Deleterious Cryptic Sequences, Facilitating the Birth of Coding Sequences.
Kosinski LJ, Masel J. Kosinski LJ, et al. Mol Biol Evol. 2020 Jun 1;37(6):1761-1774. doi: 10.1093/molbev/msaa046. Mol Biol Evol. 2020. PMID: 32101291 Free PMC article.
-
Origin of functional de novo genes in humans from "hopeful monsters".
Liu X, Xiao C, Xu X, Zhang J, Mo F, Chen JY, Delihas N, Zhang L, An NA, Li CY. Liu X, et al. Wiley Interdiscip Rev RNA. 2024 Mar-Apr;15(2):e1845. doi: 10.1002/wrna.1845. Wiley Interdiscip Rev RNA. 2024. PMID: 38605485 Review.
-
Schmitz JF, Bornberg-Bauer E. Schmitz JF, et al. F1000Res. 2017 Jan 19;6:57. doi: 10.12688/f1000research.10079.1. eCollection 2017. F1000Res. 2017. PMID: 28163910 Free PMC article. Review.
Cited by
-
Fukushima K, Pollock DD. Fukushima K, et al. Nat Commun. 2020 Sep 8;11(1):4459. doi: 10.1038/s41467-020-18090-8. Nat Commun. 2020. PMID: 32900997 Free PMC article.
-
Perochon A, Kahla A, Vranić M, Jia J, Malla KB, Craze M, Wallington E, Doohan FM. Perochon A, et al. Plant Biotechnol J. 2019 Oct;17(10):1892-1904. doi: 10.1111/pbi.13105. Epub 2019 Apr 29. Plant Biotechnol J. 2019. PMID: 30821405 Free PMC article.
-
The Cryptic Bacterial Microproteome.
Fesenko I, Sahakyan H, Shabalina SA, Koonin EV. Fesenko I, et al. bioRxiv [Preprint]. 2024 Feb 18:2024.02.17.580829. doi: 10.1101/2024.02.17.580829. bioRxiv. 2024. PMID: 38903115 Free PMC article. Preprint.
-
The origin and structural evolution of de novo genes in Drosophila.
Peng J, Zhao L. Peng J, et al. Nat Commun. 2024 Jan 27;15(1):810. doi: 10.1038/s41467-024-45028-1. Nat Commun. 2024. PMID: 38280868 Free PMC article.
-
Intergenic ORFs as elementary structural modules of de novo gene birth and protein evolution.
Papadopoulos C, Callebaut I, Gelly JC, Hatin I, Namy O, Renard M, Lespinet O, Lopes A. Papadopoulos C, et al. Genome Res. 2021 Dec;31(12):2303-2315. doi: 10.1101/gr.275638.121. Epub 2021 Nov 22. Genome Res. 2021. PMID: 34810219 Free PMC article.
References
LinkOut - more resources
Full Text Sources
Other Literature Sources