NGS-based analysis of base-substitution signatures created by yeast DNA polymerase eta and zeta on undamaged and abasic DNA templates in vitro - PubMed
NGS-based analysis of base-substitution signatures created by yeast DNA polymerase eta and zeta on undamaged and abasic DNA templates in vitro
Yizhang Chen et al. DNA Repair (Amst). 2017 Nov.
Abstract
Translesion synthesis (TLS) is the mechanism in which DNA polymerases (TLS polymerases) bypass unrepaired template damage with high error rates. DNA polymerase η and ζ (Polη and Polζ) are major TLS polymerases that are conserved from yeast to humans. In this study, we quantified frequencies of base-substitutions by yeast Polη and Polζ on undamaged and abasic templates in vitro. For accurate quantification, we used a next generation sequencing (NGS)-based method where DNA products were directly analyzed by parallel sequencing. On undamaged templates, Polη and Polζ showed distinct base-substitution profiles, and the substitution frequencies were differently influenced by the template sequence. The base-substitution frequencies were influenced mainly by the adjacent bases both upstream and downstream of the substitution sites. Thus we present the base-substitution signatures of these polymerases in a three-base format. On templates containing abasic sites, Polη created deletions at the lesion in more than 50% of the TLS products, but the formation of the deletions was suppressed by the presence of Polζ. Polζ and Polη cooperatively facilitated the TLS reaction over an abasic site in vitro, suggesting that these two polymerases can cooperate in efficient and high fidelity TLS.
Keywords: DNA damage; DNA repair; Error-prone polymerase; Mutagenesis; Mutation signature; Next generation sequencing; Translesion synthesis.
Copyright © 2017 Elsevier B.V. All rights reserved.
Conflict of interest statement
Conflict of interest statement
None declared.
Figures
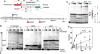
(A) Schematic drawing of Synthetic DNA substrates for TLS reactions. The NGS-primer contained an A-adaptor (red box) and a 6-nt barcode (yellow box, BC) for Ion Torrent PGM. Templates contained a P1-adaptor at the 5′-end (green box) and the complementary sequence to the primer (20-nt-long region) at the 3′ end. The product, which has both adaptors, is recognized by the NGS system that reads the sequences of the 73-nt region (position #1–73), in which the first 20-nt region (position #1–20) are part of the primer, and the remaining 53-nt region (position #21–73; variable region) is extended by yeast DNA polymerases. In addition to the template without lesion (type-A), we used templates with the same sequences but containing an AP site at the position #28 (28AP) or position #43 (43AP). All templates were modified with biotin at the 3′-OH group to prevent extension. The NGS primer had a one-base mismatch at position #10 (10G) to distinguish the primer-extension products from any template-extension product. (B) Primer extension reactions were carried out using the indicated polymerase and 32P-labeled primer (34mer) hybridized to the template with no damage, 28AP, or 43AP. After incubating for 2.5, 5, or 10 min (Polδ and ε), or 10, 20, or 40 min (Polη and ζ), a small portion was withdrawn and analyzed by denaturing polyacrylamide gel. (C) The same TLS reactions as in panel B were carried out on the template containing 43AP by Polη, Polζ, and a mixture of Polη and Polζ, and the reaction was stopped at 5, 10, 20, 40, and 80 min. (D) The TLS efficiency (% of the fully extended products in all DNA molecules that reached the AP site) was calculated from C and repeated experiments (error bar is SD (n=3)).
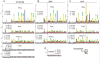
(A–C) In vitro primer extension reactions were carried out with the substrates shown in Fig. 1A, containing no lesion (A), 28AP (B), or 43AP (C), and indicated DNA polymerases, and the products were analyzed by the NGS system. Base-substitutions in the output sequences were mapped on the reference sequence after barcode sorting and quality control. The reference sequence (sequence of growing strand) is given under each graph, in which underlined region belongs to the primer. Pink dashed lines indicate the positions of AP sites. Base-substitution data at the AP sites are omitted in this figure (shown in Fig. 5). Vertical axis (%) indicates the frequency of base-substitution per synthesis at indicated position of template. Reactions were repeated three times and mean data with SD of the triplicate experiments are shown. The number of qualified output sequences that was obtained from each reaction is given as “n”. See text and Materials and Methods for more details. (D) An oligo containing two adaptors (TSO561, Supplemental Fig. S1C and Table S1) was directly subjected to NGS analysis to quantitate background of the analysis.
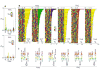
Base-substitution frequencies in total of 300-nt of undamaged template DNA, (position #22–71 of type A to F templates, constituted by 73 “A”, 74 “C”, 77 “G”, and 76 “T”) were analyzed. (A) Average base-substitution frequencies by Polη and Polζ. Error bars are SD (n are from 73 to 77). (B) The three most predominant base-substitution types by Polη and Polζ were selected from (A), and individual sites of the substitutions were sorted by frequencies. Colored squares indicate DNA sequence of the growing strand from 10-nt upstream (−10) to 5-nt downstream (+5) of the substitution sites. All substitution sites were sorted from highest (top) to lowest (bottom) frequency, which is indicated by the bar graphs. Positions of substitutions are indicated by “0”. (C) Effects of the bases from 3-nt upstream to 1-nt downstream of the substitution on the substitution frequencies. Height of each letters represents the relative substitution frequencies to the average value when the specific bases exist at given positions. Significance of difference between most and least prevalent bases at each position is indicated by asterisks (*, **, ***, and **** indicate p<0.05, p<0.01, p<0.001, and p<0.0001, respectively).
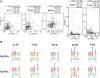
(A) Frequencies of indicated base-substitutions by Polη (x-axis) versus by Polζ (y-axis) at individual substitution sites are plotted. Error bars are SD (n=2–3). Dashed lines in the plotting area indicate medians, and numbers in four sections above each graph indicate the numbers of data points in corresponding sections in the plotting area. The value “r” is the Pearson correlation coefficient (with two-sided p values). (B) Effects of the bases from 3-nt upstream to 1-nt downstream of the substitution on the substitution frequencies by each polymerase are expressed as heights of the letters, as in Fig. 3C.
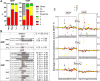
(A) Nucleotides opposite to the AP sites. TLS reaction was carried out on the templates containing 28AP or 43AP by Polη, Polζ, or a mixture of Polη and Polζ (η+ζ). The fully extended TLS products were analyzed by NGS, and the nucleotides inserted opposite to the AP sites (colored bars), single nucleotide and multi-nucleotide deletions to skip the lesion (open and grey bars, respectively), more than one nucleotide insertions over the lesion (striped bar) and others (black bar) were quantified. (B) Analysis of the multi-nucleotide deletions at AP sites. Shaded sequences indicate the sites of deletion at indicated percentages in the TLS products. The red “X” is the position opposite to the AP site. All the deletions produced at the frequencies above 0.1% in each TLS product were shown. (C) Effects of AP sites on base substitutions in the flanking regions. Base substitutions that were changed by the presence of AP sites were calculated by subtracting the substitution frequencies on the undamaged template from the frequencies on the AP-containing templates.
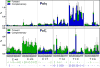
Substitution frequencies of Polη and Polζ at the center of three-nucleotide sequences were calculated from the data presented in Fig. 2A and Supplemental Fig. S2. Frequencies of complementary changes are organized in the same columns in different colors. For example, “5′ATC→5′AGC” substitution and “5′GAT→5′GCT” substitution are complementary to each other, thus their frequencies are categorized in the “T → G” group and stacked in the single column (ATC) as green (forward) and blue (complementary) bars, respectively. Asterisks below the graphs indicate significance of difference in the two polymerases making forward (green asterisks) and complementary (blue asterisks) base-substitutions (*, **, ***, and **** indicate p<0.05, p<0.01, p<0.001, and p<0.0001, respectively).
Similar articles
-
Role of DNA polymerase eta in the bypass of abasic sites in yeast cells.
Zhao B, Xie Z, Shen H, Wang Z. Zhao B, et al. Nucleic Acids Res. 2004 Jul 29;32(13):3984-94. doi: 10.1093/nar/gkh710. Print 2004. Nucleic Acids Res. 2004. PMID: 15284331 Free PMC article.
-
Kozmin SG, Pavlov YI, Kunkel TA, Sage E. Kozmin SG, et al. Nucleic Acids Res. 2003 Aug 1;31(15):4541-52. doi: 10.1093/nar/gkg489. Nucleic Acids Res. 2003. PMID: 12888515 Free PMC article.
-
Abdulovic AL, Minesinger BK, Jinks-Robertson S. Abdulovic AL, et al. DNA Repair (Amst). 2007 Sep 1;6(9):1307-18. doi: 10.1016/j.dnarep.2007.02.026. Epub 2007 Apr 17. DNA Repair (Amst). 2007. PMID: 17442629
-
Protein Assemblies in Translesion Synthesis.
Arianna GA, Korzhnev DM. Arianna GA, et al. Genes (Basel). 2024 Jun 24;15(7):832. doi: 10.3390/genes15070832. Genes (Basel). 2024. PMID: 39062611 Free PMC article. Review.
-
The Rev1-Polζ translesion synthesis mutasome: Structure, interactions and inhibition.
Rizzo AA, Korzhnev DM. Rizzo AA, et al. Enzymes. 2019;45:139-181. doi: 10.1016/bs.enz.2019.07.001. Epub 2019 Aug 9. Enzymes. 2019. PMID: 31627876 Free PMC article. Review.
Cited by
-
Phosphorylation Alters the Properties of Pol η: Implications for Translesion Synthesis.
Peddu C, Zhang S, Zhao H, Wong A, Lee EYC, Lee MYWT, Zhang Z. Peddu C, et al. iScience. 2018 Aug 31;6:52-67. doi: 10.1016/j.isci.2018.07.009. Epub 2018 Jul 18. iScience. 2018. PMID: 30240625 Free PMC article.
-
Biochemical reconstitution of heat-induced mutational processes.
Sugiyama T. Sugiyama T. PLoS One. 2024 Sep 17;19(9):e0310601. doi: 10.1371/journal.pone.0310601. eCollection 2024. PLoS One. 2024. PMID: 39288122 Free PMC article.
-
Biochemical and photochemical mechanisms that produce different UV-induced mutation spectra.
Sugiyama T, Keinard B, Best G, Sanyal MR. Sugiyama T, et al. Mutat Res. 2021 Jul-Dec;823:111762. doi: 10.1016/j.mrfmmm.2021.111762. Epub 2021 Sep 17. Mutat Res. 2021. PMID: 34563793 Free PMC article.
-
Biochemical reconstitution of UV-induced mutational processes.
Sugiyama T, Chen Y. Sugiyama T, et al. Nucleic Acids Res. 2019 Jul 26;47(13):6769-6782. doi: 10.1093/nar/gkz335. Nucleic Acids Res. 2019. PMID: 31053851 Free PMC article.
-
Sugiyama T, Sanyal MR. Sugiyama T, et al. DNA Repair (Amst). 2024 Feb;134:103617. doi: 10.1016/j.dnarep.2023.103617. Epub 2023 Dec 22. DNA Repair (Amst). 2024. PMID: 38154332 Free PMC article.
References
-
- Prakash S, Johnson RE, Prakash L. Eukaryotic translesion synthesis DNA polymerases: specificity of structure and function. Annu Rev Biochem. 2005;74:317–353. - PubMed
-
- Lindahl T. DNA repair enzymes. Annu Rev Biochem. 1982;51:61–87. - PubMed
-
- Lindahl T. Instability and decay of the primary structure of DNA. Nature. 1993;362:709–715. - PubMed
-
- Nakamura J, Swenberg JA. Endogenous apurinic/apyrimidinic sites in genomic DNA of mammalian tissues. Cancer Res. 1999;59:2522–2526. - PubMed
MeSH terms
Substances
LinkOut - more resources
Full Text Sources
Other Literature Sources
Molecular Biology Databases