Common TFIIH recruitment mechanism in global genome and transcription-coupled repair subpathways - PubMed
- ️Sun Jan 01 2017
Common TFIIH recruitment mechanism in global genome and transcription-coupled repair subpathways
Masahiko Okuda et al. Nucleic Acids Res. 2017.
Abstract
Nucleotide excision repair is initiated by two different damage recognition subpathways, global genome repair (GGR) and transcription-coupled repair (TCR). In GGR, XPC detects DNA lesions and recruits TFIIH via interaction with the pleckstrin homology (PH) domain of TFIIH subunit p62. In TCR, an elongating form of RNA Polymerase II detects a lesion on the transcribed strand and recruits TFIIH by an unknown mechanism. Here, we found that the TCR initiation factor UVSSA forms a stable complex with the PH domain of p62 via a short acidic string in the central region of UVSSA, and determined the complex structure by NMR. The acidic string of UVSSA binds strongly to the basic groove of the PH domain by inserting Phe408 and Val411 into two pockets, highly resembling the interaction mechanism of XPC with p62. Mutational binding analysis validated the structure and identified residues crucial for binding. TCR activity was markedly diminished in UVSSA-deficient cells expressing UVSSA mutated at Phe408 or Val411. Thus, a common TFIIH recruitment mechanism is shared by UVSSA in TCR and XPC in GGR.
© The Author(s) 2017. Published by Oxford University Press on behalf of Nucleic Acids Research.
Figures
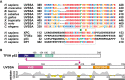
Amino acid sequence and domain organization of UVSSA. (A) Alignment of the amino acid sequences of UVSSA and the TFIIH p62 PH-binding regions of human XPC, TFIIEα, p53, and DP1. Green and orange circles above the sequence of XPC indicate the amino acids that insert into pocket 1 and pocket 2 in p62 PH, respectively. (B) Domain organization of TFIIH p62 and UVSSA. Intrinsically disordered regions in UVSSA predicted by IDEAL (55) are indicated by the black bars.
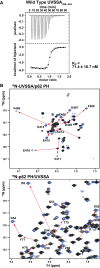
Specific binding of UVSSA to TFIIH p62 PH. (A) Isothermal titration calorimetry for the binding of UVSSA390−434 to TFIIH p62 PH. Shown are thermogram (upper panel) and binding isotherm (lower panel) of the calorimetric titration. Titrant: wild-type UVSSA390–434. (B) NMR chemical shift perturbation for 15N-labeled UVSSA390−434 with TFIIH p62 PH (upper panel) and for 15N-labeled TFIIH p62 PH with UVSSA390−434 (lower panel). Shown in overlay of 1H–15N HSQC spectra before (black) and after (blue) the addition of unlabeled sample. Selected regions are shown here (see full region in Supplementary Figures S1 and S2). Residues showing a large chemical shift change are labeled.
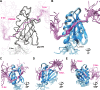
Structure of the complex between UVSSA and TFIIH p62 PH. (A) The 20 best solution structures represented as a line diagram. UVSSA and p62 are shown in magenta and black, respectively. (B–E) The 20 best solution structures represented as a ribbon diagram. UVSSA and p62 are shown in magenta and blue, respectively. For clarity, the four N-terminal residues and the nine C-terminal residues of UVSSA390–434 have been omitted. The structures in C, D, and E are rotated by 45, -45, and 180 degrees, respectively, relative to the structures in A and B.
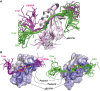
Structural comparison of the UVSSA–p62 PH and XPC–p62 PH complexes. (A) Superimposition of the best 20 structures of the UVSSA–p62 PH complex (magenta/pink) and those of the XPC–p62 PH complex (green/grey). (B) Binding surface of p62 PH in complex with UVSSA (left) and XPC (right). The electrostatic potential surfaces are shown. Positive potential is shown in blue, and negative potential in red. UVSSA and XPC are shown in stick representation (best 20 structures).
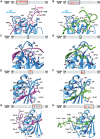
Comparison of p62 PH recognition between TCR factor UVSSA and GGR factor XPC. (A, C, E, G) Intermolecular interactions in the UVSSA–p62 PH complex. (B, D, F, H) Intermolecular interactions in the XPC–p62 PH complex. p62, UVSSA, and XPC are shown in blue, magenta, and green, respectively. To discriminate p62 from UVSSA or XPC, residues of p62 are labeled in lowercase and residues of UVSSA or XPC are labeled in uppercase.
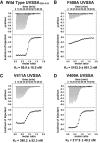
ITC analysis of p62 PH binding by UVSSA mutant. Shown are thermograms (upper panels) and binding isotherms (lower panels) of the calorimetric titration. (A) Titrant: wild type UVSSA399–419. (B) Titrant: F408A UVSSA399–419. (C) Titrant: V411A UVSSA399–419. (D) Titrant: V409A UVSSA399–419. The calculated binding dissociation constant (Kd) is shown below each panel. Data are the mean ± standard error (S.D.) from the fitting curve.
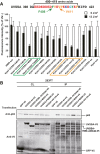
The p62 binding domain of UVSSA is essential for transcription coupled NER activity. (A) Recovery of RNA synthesis was measured in Kps3 cells expressing wild-type UVSSA–V5 or UVSSA–V5 with mutations in the p62-binding domain (filled bars, 12 J/m2 of UV-C; open bars, no UV). Aromatic (green) and hydrophobic (orange) residues required for strong binding between UVSSA and p62 are indicated. Error bars represent the standard error (S.E.) of quadrupled experiments. (B) The interaction of p62 with either wild-type UVSSA or various UVSSA mutant proteins was assayed by immunoprecipitation, followed by western blotting. CL, crude lysate; IP, immunoprecipitate.
Similar articles
-
Okuda M, Kinoshita M, Kakumu E, Sugasawa K, Nishimura Y. Okuda M, et al. Structure. 2015 Oct 6;23(10):1827-1837. doi: 10.1016/j.str.2015.07.009. Epub 2015 Aug 13. Structure. 2015. PMID: 26278177
-
Elf1 promotes transcription-coupled repair in yeast by using its C-terminal domain to bind TFIIH.
Selvam K, Xu J, Wilson HE, Oh J, Li Q, Wang D, Wyrick JJ. Selvam K, et al. Nat Commun. 2024 Jul 23;15(1):6223. doi: 10.1038/s41467-024-50539-y. Nat Commun. 2024. PMID: 39043658 Free PMC article.
-
Mistry H, Kumari S, Aswal VK, Gupta GD. Mistry H, et al. Int J Biol Macromol. 2023 Aug 30;247:125792. doi: 10.1016/j.ijbiomac.2023.125792. Epub 2023 Jul 11. Int J Biol Macromol. 2023. PMID: 37442507
-
Fuss JO, Tainer JA. Fuss JO, et al. DNA Repair (Amst). 2011 Jul 15;10(7):697-713. doi: 10.1016/j.dnarep.2011.04.028. Epub 2011 May 14. DNA Repair (Amst). 2011. PMID: 21571596 Free PMC article. Review.
-
Molecular mechanisms of DNA damage recognition for mammalian nucleotide excision repair.
Sugasawa K. Sugasawa K. DNA Repair (Amst). 2016 Aug;44:110-117. doi: 10.1016/j.dnarep.2016.05.015. Epub 2016 May 20. DNA Repair (Amst). 2016. PMID: 27264556 Review.
Cited by
-
Elongation factor ELOF1 drives transcription-coupled repair and prevents genome instability.
Geijer ME, Zhou D, Selvam K, Steurer B, Mukherjee C, Evers B, Cugusi S, van Toorn M, van der Woude M, Janssens RC, Kok YP, Gong W, Raams A, Lo CSY, Lebbink JHG, Geverts B, Plummer DA, Bezstarosti K, Theil AF, Mitter R, Houtsmuller AB, Vermeulen W, Demmers JAA, Li S, van Vugt MATM, Lans H, Bernards R, Svejstrup JQ, Ray Chaudhuri A, Wyrick JJ, Marteijn JA. Geijer ME, et al. Nat Cell Biol. 2021 Jun;23(6):608-619. doi: 10.1038/s41556-021-00692-z. Epub 2021 Jun 9. Nat Cell Biol. 2021. PMID: 34108662 Free PMC article.
-
Gonzalo-Hansen C, Steurer B, Janssens RC, Zhou D, van Sluis M, Lans H, Marteijn JA. Gonzalo-Hansen C, et al. Nucleic Acids Res. 2024 Sep 9;52(16):9596-9612. doi: 10.1093/nar/gkae618. Nucleic Acids Res. 2024. PMID: 39021334 Free PMC article.
-
The Dark Side of UV-Induced DNA Lesion Repair.
Strzałka W, Zgłobicki P, Kowalska E, Bażant A, Dziga D, Banaś AK. Strzałka W, et al. Genes (Basel). 2020 Dec 2;11(12):1450. doi: 10.3390/genes11121450. Genes (Basel). 2020. PMID: 33276692 Free PMC article. Review.
-
STK19 positions TFIIH for cell-free transcription-coupled DNA repair.
Mevissen TET, Kümmecke M, Schmid EW, Farnung L, Walter JC. Mevissen TET, et al. bioRxiv [Preprint]. 2024 Jul 23:2024.07.22.604623. doi: 10.1101/2024.07.22.604623. bioRxiv. 2024. PMID: 39091863 Free PMC article. Updated. Preprint.
-
Transcription-coupled nucleotide excision repair: New insights revealed by genomic approaches.
Duan M, Speer RM, Ulibarri J, Liu KJ, Mao P. Duan M, et al. DNA Repair (Amst). 2021 Jul;103:103126. doi: 10.1016/j.dnarep.2021.103126. Epub 2021 Apr 20. DNA Repair (Amst). 2021. PMID: 33894524 Free PMC article. Review.
References
-
- Lehmann A.R. DNA repair-deficient diseases, xeroderma pigmentosum, Cockayne syndrome and trichothiodystrophy. Biochimie. 2003; 85:1101–1111. - PubMed
-
- Naegeli H., Sugasawa K.. The xeroderma pigmentosum pathway: decision tree analysis of DNA quality. DNA Repair. 2011; 10:673–683. - PubMed
-
- Fousteri M., Mullenders L.H.. Transcription-coupled nucleotide excision repair in mammalian cells: molecular mechanisms and biological effects. Cell Res. 2008; 18:73–84. - PubMed
-
- Hanawalt P.C., Spivak G.. Transcription-coupled DNA repair: two decades of progress and surprises. Nat. Rev. Mol. Cell Biol. 2008; 9:958–970. - PubMed
MeSH terms
Substances
LinkOut - more resources
Full Text Sources
Other Literature Sources
Research Materials