Vibrational Detection of Odorant Functional Groups by Drosophila melanogaster - PubMed
- ️Sun Jan 01 2017
Vibrational Detection of Odorant Functional Groups by Drosophila melanogaster
Klio Maniati et al. eNeuro. 2017.
Abstract
A remarkable feature of olfaction, and perhaps the hardest one to explain by shape-based molecular recognition, is the ability to detect the presence of functional groups in odorants, irrespective of molecular context. We previously showed that Drosophila trained to avoid deuterated odorants could respond to a molecule bearing a nitrile group, which shares the vibrational stretch frequency with the CD bond. Here, we reproduce and extend this finding by showing analogous olfactory responses of Drosophila to the chemically vastly different functional groups, thiols and boranes, that nevertheless possess a common vibration at 2600 cm-1. Furthermore, we show that Drosophila do not respond to a cyanohydrin structure that renders nitrile groups invisible to IR spectroscopy. We argue that the response of Drosophila to these odorants which parallels their perception in humans, supports the hypothesis that odor character is encoded in odorant molecular vibrations, not in the specific shape-based activation pattern of receptors.
Keywords: Drosophila; functional groups; olfaction; vibrations.
Figures
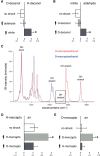
Odorant vibrations guide conditioned behavior in Drosophila. All graphs represent the mean relative distribution of flies in the arms of the maze ± SEM after the indicated treatment. The odorants or air converging in the center of the maze during the test phase, are indicated above each graph. “No shock” condition refers to groups of naïve flies responding to the indicated odor(s). Other groups of flies were trained by repeated electric footshocks (lightning bolts) in the presence of the indicated odorants per condition. All significance values are calculated relative to the performance of naïve animals (open bars), unless noted otherwise, by LSM contrast analysis and are indicated on the graphs with a single star. A, Transfer of olfactory learning between citronellyl nitrile (nitrile) and D-decanol. Initial ANOVA indicated significant differences (F(2,40) = 5.5868, p < 0.007). Flies trained to avoid citronellal (aldehyde) did not significantly avoid (p = 0.4267) either test odorant (light gray bar), whereas flies shocked against nitrile (dark gray bar) avoided D-decanol significantly (p = 0.003). n > 10 for all conditions, with a total of 700–900 flies per condition. B, In the converse experiment, ANOVA indicated significant differences (F(2,38) = 4.8303, p < 0.01). Subsequent contrast analysis revealed that flies trained to avoid H-decanol did not change their spontaneous behavior (light gray bar, p = 0.5029). In contrast, animals trained to avoid D-decanol presented significant avoidance to the nitrile (dark gray bar, p = 0.0073). n > 10 for all conditions, with a total of 700–1000 flies per condition. C, Computed I.R spectra of H- and D4-β-mercaptoethanol indicating the frequencies of the relevant C–D, -SH, and C–H stretches. D, Conditioned H- and D4-β-mercaptoethanol discrimination. Initial ANOVA indicated significant differences (F(2,43) = 6.2144, p < 0.004). Flies trained to selectively avoid the H isotopologue augmented their avoidance of H-β-mercaptoethanol over that of naïve animals (p = 0.0041), but not for D4-β-mercaptoethanol (p = 0.9536). The performances of the two groups trained to different isotopologues were also significantly different (p = 0.0040). n ≥ 14 for all conditions, with a total of 900–1000 flies per condition. E, In the converse experiment, animals trained with each of the isotopologues were tested against D-β-mercaptoethanol. ANOVA indicated significant differences (F(2,31) = 3.6338, p < 0.03). Animals trained with the D isotopologue enhanced their avoidance of D-β-mercaptoethanol (p = 0.0259), but not of H-β-mercaptoethanol (p = 0.9236). The performance of the two groups is different (p = 0.0255). n > 10 for all conditions, with a total of 600–700 flies per condition.
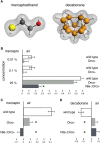
Responses to β-mercaptoethanol and decaborane in wild-type Orco− and Ir8a− Orco− double mutants. A, The high molecular divergence of β-mercaptoethanol and decaborane. B–D, All graphs represent the mean relative distribution of flies in the arms of the maze ± SEM after the indicated treatment. Groups of flies were exposed to the indicated odorants per condition. Significance values are calculated by LSM contrast analysis and are indicated on the graphs with a single star. B, Responses of wild-type and anosmic flies to a range of β-mercaptoethanol concentrations. Wild-type flies are shown in white bars, Orco− flies are shown in light gray bars and Ir8a;Orco- flies are shown in dark gray bars. ANOVA indicated significant differences (F(6,87) = 39.7679, p < 0.0001). At the 0.01% concentration, the difference between wild-type and Orco− flies is not significant (p = 0.2394), but at the 0.1% concentration, the difference is significant (p = 0.0454). At the 25% concentration the response of wild-type and Orco− flies is not significantly different (p = 0.1938) but that of Ir8a−;Orco−is (p < 0.0001). n ≥ 8 for all conditions, with a total of 600-1000 flies per condition. C, ANOVA indicated significant differences (F(2,35) = 38.3487, p < 0.0001), which were revealed due to the significant difference in avoidance of β-mercaptoethanol by wild-type and both Orco− and Ir8a;Orco- mutants (both p < 0.0001). The difference between Orco− and Ir8a;Orco- mutants is also significant (p = 0.0445). n ≥ 10 for all conditions, with a total of 700-900 flies per condition. D, ANOVA indicated significant differences (F(2,62) = 2.5955, p < 0.0830), underlying the significant difference in attraction to decaborane between wild-type, Orco− mutants (p = 0.0518), and Ir8a;Orco- (p = 0.0341). The difference between Orco− and Ir8a;Orco- mutants is not significant (p = 0.8832). n ≥ 12 for all conditions, with a total of 800–1400 flies per condition.
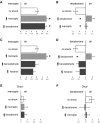
After conditioning Drosophila do not discriminate decaborane from β-mercaptoethanol. All graphs represent the mean relative distribution of flies in the arms of the maze ± SEM after the indicated treatment. Significant differences are calculated relative to the performance of naïve animals (open bars) by LSM contrast analysis and are indicated on the graphs with a single star. A, ANOVA indicated significant differences (F(2,59) = 8.5292, p = 0.0006). Flies shocked in the presence of β-mercaptoethanol augmented their avoidance toward it (light gray bar, p = 0.0005) and surprisingly a similar augmentation was shown by animals shocked in the presence of decaborane (dark bar, p = 0.0006). Indeed, the performances of the two differently trained groups were not significantly different (p = 0.5915). n ≥ 12 for all conditions, with a total of 900-2000 flies per condition. B, In the converse experiment ANOVA indicated significant differences (F(2,30) = 15.3343, p < 0.0001). Flies shocked in the presence of decaborane avoid it instead of being attracted to it as naïve animals do (light gray bar, p < 0.0001) and a similar response is observed in β-mercaptoethanol-trained animals (dark bar, p = 0.0006). The two trained groups do not show significantly different responses (p = 0.1150). n ≥ 8 for all conditions, with a total of 500–900 flies per condition. C, Animals shocked against benzaldehyde (10%) or (10%) hexanol do not change their spontaneous response to β-mercaptoethanol. ANOVA indicated significant differences (F(3,60) = 7.9061, p = 0.0002). Subsequent contrast analysis revealed significant differences in the performance of β-mercaptoethanol-trained animals (light gray bars) versus those trained with benzaldehyde (p = 0.0006) and hexanol (p < 0.0001). The responses of flies trained with benzaldehyde and hexanol (dark bars) were not significantly different (p = 0.3997 and p = 0.0813, respectively) from naïve animals (open bars). n ≥ 9 for all conditions, with a total of 700–2000 flies per condition. D, Animals shocked against benzaldehyde or hexanol do not change their spontaneous response to decaborane. ANOVA indicated significant differences (F(3,43) = 4.9331, p = 0.0052). Contrast analysis revealed significant differences in the performance of decaborane-trained animals (light gray bars) versus those trained with benzaldehyde (p = 0.0003) and hexanol (p = 0.0004). The responses of flies trained with benzaldehyde and hexanol (dark bars) were not significantly different (p = 0.2403 and p = 0.1561, respectively) from naïve animals (open bars). n ≥ 8 for all conditions, with a total of 500–900 flies per condition. E, ANOVA did not indicate significant differences (F(2,26) = 0.1105, p = 0.8959). Anosmic animals shocked in the presence of β-mercaptoethanol or in the presence of decaborane did not augment their response to either (light gray bar, p = 0.6792 and dark gray bar, p = 0.7059, respectively). Furthermore, the performances of the two differently trained groups of flies were not significantly different (p = 0.9624). n ≥ 8 for all conditions, with a total of 600-700 flies per condition. F, In the converse experiment, ANOVA also did not indicate significant differences (F(2,48) = 0.7551, p = 0.4757). Flies shocked in the presence of decaborane or β-mercaptoethanol did not change their irresponsiveness toward decaborane (light gray bar, p =0.24 and dark bar, p = 0.42, respectively). The responses of the two differently trained groups were not significantly different (p = 0.69). n ≥ 8 for all conditions, with a total of 800–1000 flies per condition.
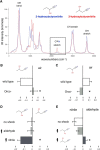
Differential responses to nitrile in the context of different hydroxynitriles. A, Computed I.R spectra of 2- and 3-hydroxybutyronitrile. The location of the -OH group that changes the intensity of the -C≡N stretch (boxed) is shown. Graphs represent the mean relative distribution of flies in the arms of the maze ± SEM after the indicated treatment per condition. Groups of flies were trained by repeated electric footshocks (lightning bolts) in the presence of the indicated odorants per condition. All significance values are calculated relative to the performance of naïve animals (open bars) by LSM contrast analysis and are indicated on the graphs with a single star. B, ANOVA indicated significant differences (F(1,19) = 7.6564, p < 0.012), underlying the significant difference (p = 0.0076) in attraction to 3-hydroxybutyronitrile by wild-type and Orco− mutants. n ≥ 7 for all conditions, with a total of 400-500 flies per condition. C, ANOVA indicated significant differences (F(3,39) = 6014, p < 0.002), underlying the significant difference (p = 0.0136) in avoidance of 2-hydroxybutyronitrile by wild-type and Orco− mutants. n ≥ 11 for all conditions, with a total of 700-800 flies per condition. D, ANOVA indicated significant differences (F(2,39) = 3.4240, p < 0.04), when flies were differentially trained with citronellal (aldehyde). Flies trained with the aldehyde did not respond differentially (light gray bar, p = 0.9052) when tested against the two hydroxynitriles. In contrast, animals trained with citronellyl nitrile (nitrile) avoided preferentially 3-hydroxybutyronitrile (dark bar, p = 0.0208). The performance of the differently trained groups was significantly different (p = 0.0439). n ≥ 11 for all conditions, with a total of 600-900 flies per condition. E, In the complementary experiment ANOVA indicated significant differences (F(2,24) = 11.3598, p < 0.0004). Flies trained with 2-hydroxybutyronitrile did not exhibit responses different from naïve flies (light gray bar, p= 0.1993). However, flies shocked in the presence of 3-hydroxybutyronitrile avoided the nitrile significantly (dark gray bar, p = 0.0001). The performance of the two differently trained groups was significantly different (p = 0.0058). n ≥ 11 for all conditions, with a total of 500-600 flies per condition.
Similar articles
-
Molecular vibration-sensing component in Drosophila melanogaster olfaction.
Franco MI, Turin L, Mershin A, Skoulakis EM. Franco MI, et al. Proc Natl Acad Sci U S A. 2011 Mar 1;108(9):3797-802. doi: 10.1073/pnas.1012293108. Epub 2011 Feb 14. Proc Natl Acad Sci U S A. 2011. PMID: 21321219 Free PMC article.
-
Differential Electrophysiological Responses to Odorant Isotopologues in Drosophilid Antennae.
Drimyli E, Gaitanidis A, Maniati K, Turin L, Skoulakis EM. Drimyli E, et al. eNeuro. 2016 Jun 20;3(3):ENEURO.0152-15.2016. doi: 10.1523/ENEURO.0152-15.2016. eCollection 2016 May-Jun. eNeuro. 2016. PMID: 27351023 Free PMC article.
-
Molecular vibration-sensing component in human olfaction.
Gane S, Georganakis D, Maniati K, Vamvakias M, Ragoussis N, Skoulakis EM, Turin L. Gane S, et al. PLoS One. 2013;8(1):e55780. doi: 10.1371/journal.pone.0055780. Epub 2013 Jan 25. PLoS One. 2013. PMID: 23372854 Free PMC article.
-
Odor and pheromone detection in Drosophila melanogaster.
Smith DP. Smith DP. Pflugers Arch. 2007 Aug;454(5):749-58. doi: 10.1007/s00424-006-0190-2. Epub 2007 Jan 5. Pflugers Arch. 2007. PMID: 17205355 Review.
-
Engineering Aspects of Olfaction.
Persaud KC. Persaud KC. In: Persaud KC, Marco S, Gutiérrez-Gálvez A, editors. Neuromorphic Olfaction. Boca Raton (FL): CRC Press/Taylor & Francis; 2013. Chapter 1. In: Persaud KC, Marco S, Gutiérrez-Gálvez A, editors. Neuromorphic Olfaction. Boca Raton (FL): CRC Press/Taylor & Francis; 2013. Chapter 1. PMID: 26042329 Free Books & Documents. Review.
Cited by
-
Platelet olfactory receptor activation limits platelet reactivity and growth of aortic aneurysms.
Morrell CN, Mix D, Aggarwal A, Bhandari R, Godwin M, Owens P 3rd, Lyden SP, Doyle A, Krauel K, Rondina MT, Mohan A, Lowenstein CJ, Shim S, Stauffer S, Josyula VP, Ture SK, Yule DI, Wagner LE 3rd, Ashton JM, Elbadawi A, Cameron SJ. Morrell CN, et al. J Clin Invest. 2022 May 2;132(9):e152373. doi: 10.1172/JCI152373. J Clin Invest. 2022. PMID: 35324479 Free PMC article.
-
Quantum tunnelling in the context of SARS-CoV-2 infection.
Adams B, Sinayskiy I, van Grondelle R, Petruccione F. Adams B, et al. Sci Rep. 2022 Oct 8;12(1):16929. doi: 10.1038/s41598-022-21321-1. Sci Rep. 2022. PMID: 36209224 Free PMC article.
References
MeSH terms
LinkOut - more resources
Full Text Sources
Other Literature Sources
Molecular Biology Databases