The biology and polymer physics underlying large-scale chromosome organization - PubMed
Review
The biology and polymer physics underlying large-scale chromosome organization
Shelley Sazer et al. Traffic. 2018 Feb.
Abstract
Chromosome large-scale organization is a beautiful example of the interplay between physics and biology. DNA molecules are polymers and thus belong to the class of molecules for which physicists have developed models and formulated testable hypotheses to understand their arrangement and dynamic properties in solution, based on the principles of polymer physics. Biologists documented and discovered the biochemical basis for the structure, function and dynamic spatial organization of chromosomes in cells. The underlying principles of chromosome organization have recently been revealed in unprecedented detail using high-resolution chromosome capture technology that can simultaneously detect chromosome contact sites throughout the genome. These independent lines of investigation have now converged on a model in which DNA loops, generated by the loop extrusion mechanism, are the basic organizational and functional units of the chromosome.
Keywords: Hi-C; SMC; chromosome evolution; chromosome territory; chromosome tethering; cohesin; fractal globule; loop extrusion; polymer physics; topologically associated domains.
© 2017 The Authors. Traffic published by John Wiley & Sons Ltd.
Figures
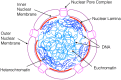
The human cell nucleus. The nucleus of all eukaryotic cells is bounded by a double phospholipid membrane (purple) composed of an inner and outer leaflet. The nuclear envelope forms a physical barrier between the chromosomes (blue) and the cytoplasm, a structural scaffold for the nucleus, and a permeability barrier between the nucleoplasm and the cytoplasm. It is perforated by nuclear pore complexes (pink) through which small molecules diffuse, and larger molecules are selectively transported. In human cells, the inner nuclear membrane is lined by the nuclear lamina (red) to which heterochromatin (dark blue) and specific chromosome domains are anchored, whereas euchromatin (light blue) is enriched in the nuclear interior21
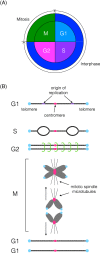
The eukaryotic cell and chromosome cycle: (A) The eukaryotic cell cycle is divided into mitosis and interphase, and interphase is further subdivided into G1, S and G2. DNA is duplicated in S phase, the chromosomes condense and segregate in M phase, and these phases are separated by Gap (growth) phases called G1 and G2. (B) Each chromosome has telomeres (blue) at its ends, a centromere (pink), to which spindle microtubules attach at M phase, and multiple origins of DNA replication (purple). In S and G2, the replicated chromosomes are held together by cohesin (green). At M, the chromosomes condense, align at the metaphase plate, individualize and are separated from one another by the mitotic spindle after cohesin release. The centromeres are at the leading edge of this mitotic chromosome movement, the telomeres trail behind, and some cell types retain this polarized positioning, called the Rabl orientation in G1, even after chromosome decondensation23, 24
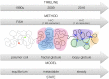
Paradigm shifts in chromatin organization. Fluorescent in situ hybridization experiments (Figure 6) on chromosomes in the 1990s suggested that DNA conformations in interphase chromosomes behave like random polymer coils at equilibrium. Chromosome conformation capture (specifically Hi‐C data at 1 Mb resolution) suggested in 2009 that the chromosomes are in a metastable polymer state, the fractal or crumbled globule. In addition, it mapped 2 sub‐compartments (indicated here by colors). More recently, Hi‐C experiments at 1 kb resolution point toward a loopy globule state, a steady state maintained by the continuous action of molecular motors called loop extrusion complexes. About 6 different sub‐compartments have been identified (3 of which are indicated here by colors, see main text for details)
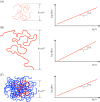
Ideal and swollen polymer coils follow different scaling laws. (A) An infinitely thin ideal polymer chain behaves like a random walk with an overall size that scales like aN 1/2 (“size” here means a quantity with units of length like the end‐to‐end distance or other related quantities that capture the overall extension of the polymer; “scales like” means that when the logarithm of the dimensionless size R/a is plotted vs the logarithm of the monomer number, N, for polymers of different degrees of polymerization the data points would lie along a line of slope 1/2 (see the right plot); a numerical prefactor in front of aN 1/2 does not affect this slope and is thus disregarded, that is, it does not matter whether R/a = N 1/2 or R/a = 10N 1/2, only that R/a ~ N 1/2). (B) The monomers of a real polymer occupy space and this excluded volume leads to a swelling of the chain to a size that scales like aN 3/5. (C) A real polymer in a dense solution of other polymers behaves like an ideal chain (compare (C) and (A)). The reason is that the outward pointing pressure produced by the monomers of the red chain is canceled by an inward pointing pressure of the other chains, shown in blue

Polymer coils are self‐similar. (A) A polymer of length aN with a stretch of length ag marked in red. (B) For an ideal chain the overall coil size scales like aN 1/2 and the size of the piece shown in red also shows the same scaling law, namely ag 1/2. Note that the polymers in (A) and (B) are shown on a different scales ((B) is magnified about 5‐fold relative to (A))
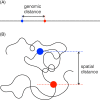
Fluorescent in situ hybridization (FISH) can be used to determine the positions of fluorescently labeled genomic loci within the nucleus. The principles of the Southern Blot in which a specific chromosomal locus is detected in vitro by hybridization to a complementary stretch of radioactively labeled DNA of known DNA sequence, were adapted for the detection of specific DNA motifs in intact cells. This in situ hybridization (ISH) technique was subsequently redesigned to use fluorescent reporters for FISH analysis. (A) A DNA polymer (chromosome) with a stretch whose ends are tagged with blue and red fluorochromes. The distance between the fluorochromes along the chain is called the chemical or genomic distance. (B) The DNA polymer (chromosome) diagrammed in (A) within a fixed cell in which the spatial distance of the 2 loci relative to nuclear landmarks or to one another can be measured. Note that the polymers in (A) and (B) are shown on different scales ((B) is magnified about 5‐fold relative to (A))
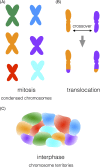
Chromosome painting identifies individual mitotic chromosomes, monitors chromosome translocations and maps chromosome territories. Chromosome painting is a technique in which chromosome‐specific fluorescently labeled DNA probes are hybridized to chromosomes and visualized using fluorescence microscopy. Chromosome painting identifies (A) individual mitotic chromosomes, (B) chromosome translocations that result from recombination crossovers between chromosomes and (C) decondensed interphase chromosome territories
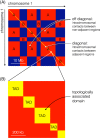
Schematic chromosome contact map of a chromosome (“chromosome 1”) at 2 levels of resolution. (A) Hi‐C data at low resolution (eg, 1 Mb resolution) display a checkerboard pattern with regions of higher probability of contact (red) and lower probability of contact (blue). This suggests the existence of 2 types of compartments, A and B, as indicated. (B) Hi‐C at higher resolution (eg, 1 kb) makes it possible to zoom in on the diagonal and reveal topologically associated domains (TADs; yellow squares) with a high probability of intradomain contact (yellow) that are physically insulated from the rest of the chromosome7
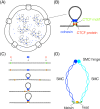
The loop extrusion model of interphase chromosome organization. (A) Decondensed interphase chromosomes territories within which the DNA is organized into loopy globules composed of topologically associated domain loops. (B) In humans and other bilaterians, cohesin (blue) and CTCF proteins (red) bound to convergent CTCF binding sites (green) localize at the base of TAD loops.87 (C) According to the loop extrusion model, 2 linked cohesins (or possibly a single cohesin) associate with DNA, form and enlarge a DNA loop until they encounter CTCF proteins bound to convergent CTCF binding motifs. Note that alternative models have been proposed and there is no known mechanism by which SMC family proteins form and enlarge chromosome loops.88, 89 Whether cohesin binds as a single complex or 2 linked complexes and the mechanism by which cohesin associates with and then dissociates from DNA are also unknown.90 (d) SMC protein complexes in eukaryotes (cohesin, condensin, SMC5/6) and prokaryotes are tripartite ring‐shaped ATPases, composed of 2 SMC proteins, (light blue, dark blue), and a Kleisin linker protein (green) that joins their head domains and assembles the nucleotide binding domain, and to which one of a family of HEAT protein regulators (yellow) bind.91 Although they share a common architecture, these complexes vary in composition (SMCs are heterodimers in eukaryotes but homodimers in prokaryotes) and biological function, which may be influenced by the Kleisin subunit and/or the Kleisin‐associated HEAT‐family regulatory component91
Similar articles
-
Genome organization via loop extrusion, insights from polymer physics models.
Ghosh SK, Jost D. Ghosh SK, et al. Brief Funct Genomics. 2020 Mar 23;19(2):119-127. doi: 10.1093/bfgp/elz023. Brief Funct Genomics. 2020. PMID: 31711163
-
Chromosome organization by one-sided and two-sided loop extrusion.
Banigan EJ, van den Berg AA, Brandão HB, Marko JF, Mirny LA. Banigan EJ, et al. Elife. 2020 Apr 6;9:e53558. doi: 10.7554/eLife.53558. Elife. 2020. PMID: 32250245 Free PMC article.
-
A mechanism of cohesin-dependent loop extrusion organizes zygotic genome architecture.
Gassler J, Brandão HB, Imakaev M, Flyamer IM, Ladstätter S, Bickmore WA, Peters JM, Mirny LA, Tachibana K. Gassler J, et al. EMBO J. 2017 Dec 15;36(24):3600-3618. doi: 10.15252/embj.201798083. Epub 2017 Dec 7. EMBO J. 2017. PMID: 29217590 Free PMC article.
-
Three-dimensional organization and dynamics of the genome.
Szalaj P, Plewczynski D. Szalaj P, et al. Cell Biol Toxicol. 2018 Oct;34(5):381-404. doi: 10.1007/s10565-018-9428-y. Epub 2018 Mar 22. Cell Biol Toxicol. 2018. PMID: 29568981 Free PMC article. Review.
-
Principles of Chromosome Architecture Revealed by Hi-C.
Eagen KP. Eagen KP. Trends Biochem Sci. 2018 Jun;43(6):469-478. doi: 10.1016/j.tibs.2018.03.006. Epub 2018 Apr 21. Trends Biochem Sci. 2018. PMID: 29685368 Free PMC article. Review.
Cited by
-
Structural and Dynamical Signatures of Local DNA Damage in Live Cells.
Eaton JA, Zidovska A. Eaton JA, et al. Biophys J. 2020 May 5;118(9):2168-2180. doi: 10.1016/j.bpj.2019.10.042. Epub 2019 Nov 13. Biophys J. 2020. PMID: 31818467 Free PMC article.
-
The self-stirred genome: large-scale chromatin dynamics, its biophysical origins and implications.
Zidovska A. Zidovska A. Curr Opin Genet Dev. 2020 Apr;61:83-90. doi: 10.1016/j.gde.2020.03.008. Epub 2020 Jun 1. Curr Opin Genet Dev. 2020. PMID: 32497955 Free PMC article. Review.
-
The rich inner life of the cell nucleus: dynamic organization, active flows, and emergent rheology.
Zidovska A. Zidovska A. Biophys Rev. 2020 Oct;12(5):1093-1106. doi: 10.1007/s12551-020-00761-x. Epub 2020 Oct 16. Biophys Rev. 2020. PMID: 33064286 Free PMC article. Review.
-
A Polymer Model of the Whole Genome.
Schiessel H. Schiessel H. Biophys J. 2020 Nov 3;119(9):1699-1700. doi: 10.1016/j.bpj.2020.09.010. Epub 2020 Sep 21. Biophys J. 2020. PMID: 33010235 Free PMC article. No abstract available.
-
Circuit Topology Analysis of Polymer Folding Reactions.
Heidari M, Schiessel H, Mashaghi A. Heidari M, et al. ACS Cent Sci. 2020 Jun 24;6(6):839-847. doi: 10.1021/acscentsci.0c00308. Epub 2020 May 12. ACS Cent Sci. 2020. PMID: 32607431 Free PMC article. Review.
References
-
- De Gennes P‐G. Scaling Concepts in Polymer Physics. Ithaca: Cornell University Press; 1979.
-
- Solovei I, Thanisch K, Feodorova Y. How to rule the nucleus: divide et impera. Curr Opin Cell Biol. 2016;40:47–59. - PubMed
Publication types
MeSH terms
Substances
LinkOut - more resources
Full Text Sources
Other Literature Sources