Hippocampus and Entorhinal Cortex Recruit Cholinergic and NMDA Receptors Separately to Generate Hippocampal Theta Oscillations - PubMed
- ️Sun Jan 01 2017
Hippocampus and Entorhinal Cortex Recruit Cholinergic and NMDA Receptors Separately to Generate Hippocampal Theta Oscillations
Zhenglin Gu et al. Cell Rep. 2017.
Abstract
Although much progress has been made in understanding type II theta rhythm generation under urethane anesthesia, less is known about the mechanisms underlying type I theta generation during active exploration. To better understand the contributions of cholinergic and NMDA receptor activation to type I theta generation, we recorded hippocampal theta oscillations from freely moving mice with local infusion of cholinergic or NMDA receptor antagonists to either the hippocampus or the entorhinal cortex (EC). We found that cholinergic receptors in the hippocampus, but not the EC, and NMDA receptors in the EC, but not the hippocampus, are critical for open-field theta generation and Y-maze performance. We further found that muscarinic M1 receptors located on pyramidal neurons, but not interneurons, are critical for cholinergic modulation of hippocampal synapses, theta generation, and Y-maze performance. These results suggest that hippocampus and EC neurons recruit cholinergic-dependent and NMDA-receptor-dependent mechanisms, respectively, to generate theta oscillations to support behavioral performance.
Keywords: NMDA receptor; Y-maze; cholinergic receptor; entorhinal cortex; hippocampus; medial septum; muscarinic; theta.
Published by Elsevier Inc.
Figures
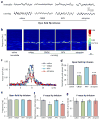
(a) Representative hippocampal local field potential recordings from one mouse freely moving in an open field arena over four trials under various intra-hippocampal antagonist infusion treatments. (b) Spectrogram analysis of representative in vivo hippocampal field potential recordings (20s) showing strong theta power during running. Theta power was impaired by hippocampal infusion of either the AMPA receptor antagonist CNQX, or the muscarinic blocker atropine, but not by the NMDA receptor antagonist APV. (c) Power spectral density analysis of representative recordings showing the power profile after various treatments. (d) Bar graph showing normalized peak theta power after infusion of different receptor antagonists to ipsilateral hippocampus. *** p = 0.0004 (CNQX), 0.0006 (APV), and 0.1742 (atropine) compared with saline treatment, n = 6 mice per treatment group, one-way ANOVA, Holm-Sidak post hoc test. (e) Bar graph showing that peak theta frequency was not significantly changed by any antagonist treatments. p = 0.9714 between groups, n = 6 mice per group, one-way ANOVA. (f) Bar graph showing that Y-maze spontaneous alternation task performance was impaired by hippocampal infusion of either CNQX or atropine, but not by APV. * p = 0.0112 for CNQX and 0.0153 for atropine compared with saline treatment, n = 7 mice per treatment group, one-way ANOVA, Holm-Sidak post hoc test. (g) Bar graph showing that the total number of Y-maze arm entries was not significantly changed over the four treatments. p = 0.9989 between treatment groups, n = 7 mice per group, one-way ANOVA.
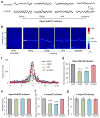
(a) Representative hippocampal local field potential recordings from one mouse freely moving in an open field arena over four trials under various intra-EC antagonist infusion treatments. (b) Spectrogram analysis of representative in vivo hippocampal field potential recordings (20s) showing that the theta power during running was impaired by EC infusion of either the AMPA receptor antagonist CNQX or by the NMDA receptor antagonist APV, but not by the muscarinic blocker atropine. (c) Power spectral density analysis of representative recordings showing the power profile after various treatments. (d) Bar graph showing normalized peak theta power after infusion of different receptor antagonists to ipsilateral EC. *** p = 0.0004 (CNQX), 0.0002 (APV), and 0.2066 (atropine) compared with saline treatment, n = 6 mice per treatment group, one-way ANOVA, Holm-Sidak post hoc test. (e) Bar graph showing that theta frequency was not significantly changed by any antagonist treatments. p = 0.6856 between groups, n = 6 mice per group, one-way ANOVA. (f) Bar graph showing that Y-maze spontaneous alternation task was impaired by EC infusion of either CNQX or APV, but not by atropine. *** p = 0.0005 for CNQX, 0.0004 for APV, and 0.7307 for atropine compared with saline treatment, n = 7 mice per treatment group, one-way ANOVA, Holm-Sidak post hoc test. (g) Bar graph showing that the total number of Y-maze arm entries was not significantly changed over the four treatments. p = 0.992 between treatments, n = 7 mice per group, one-way ANOVA.
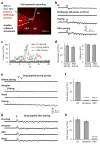
(a) The scheme of in vitro induction of theta-like oscillations in slice co-cultures. Field potentials were recorded from CA1. The Schaffer collateral (SC) pathway was activated by a stimulating electrode. Cholinergic neurons were activated via channelrhodopsin-2 that was specifically expressed in ChAT-positive neurons. (b) Induction of theta-like oscillations by paring SC and cholinergic activation, but not by either pathway activation alone. After 5–10 pairings, SC stimulation alone could then induce theta oscillations. (c) Power spectral density analysis of representative traces before, during, and 30 min after the pairing showing the peak power around 8.5 Hz. (d) Bar graphs showing similar peak power spectral density (43.2 ± 6.2 μV2/Hz at 30 min after pairing vs. 47.0 ± 7.6 μV2/Hz during pairing, n = 6 slices for each group, p = 0.70, t-test) and frequency (8.4 ± 0.35 Hz at 30 min after pairing vs. 8.6 ± 0.41 Hz during pairing, n=6 slices for each group, p = 0.71, t-test) during pairing and 30 min after the pairing. (e) Representative traces showing that theta induction was blocked by bath-applied atropine. (f) Bar graph of the peak power spectral density showing that the theta induction was blocked by either atropine or APV. **** p < 0.0001 compared with control group, n = 6 slices per group, one-way ANOVA, Holm-Sidak post hoc test. (g,h) Representative traces and bar graph showing that the theta expression was blocked by APV but not by atropine. **** p < 0.0001 compared with control, n = 5 slices for each treatment, n = 8 slices for control groups, one-way ANOVA, Holm-Sidak post hoc test.
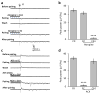
(a, b) Perfusion of atropine to CA1 but not EC before pairing blocked the induction of theta oscillations. (c, d) Perfusion of ACh to CA1 but not EC induced theta oscillations when paired with SC stimulation. **** p < 0.0001 compared with control, n = 5 slices for each group, one-way ANOVA, Holm-Sidak post hoc test.
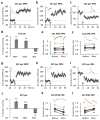
(a) Normalized SC-evoked whole cell EPSP responses from CA1 pyramidal neurons showing that cholinergic pairing (at the time of 0 min as indicated by the arrow) increased SC-evoked EPSP amplitude and the effect lasted at least 30 min after the pairing. (b) Normalized SC-evoked EPSC responses from CA1 pyramidal neurons showing that cholinergic pairing persistently increased EPSC amplitude. (c) Normalized SC-evoked IPSC responses from CA1 pyramidal neurons showing that cholinergic pairing persistently decreased IPSC amplitude. (d) Bar graph showing that cholinergic pairing significantly increased SC to CA1 synaptic EPSP amplitude (**p = 0.004, n = 6 slices, t-test) and EPSC (**p = 0.008, n = 6 slices, t-test) while it decreased IPSC amplitude (***p < 0.001, n = 6 slices, t-test). (e,f) Paired-pulse (100-ms interval) responses showing that cholinergic pairing did not significantly change the paired-pulse ratios for SC-evoked EPSP (p = 0.79, n = 7 slices, paired t-test) or IPSC responses (p = 0.83, n = 8 slices, paired t-test) in CA1. (g–j) Similar plots for EC V pyramidal neurons as in a–d for CA1 pyramidal neurons. (g) Normalized SC-evoked EPSP responses from EC V pyramidal neurons showing that cholinergic pairing persistently increased EPSP amplitude. (h) Normalized SC-evoked EPSC responses from EC V pyramidal neurons showing that cholinergic pairing persistently increased EPSC amplitude. (i) Normalized SC-evoked IPSC responses from EC V pyramidal neurons showing that cholinergic pairing persistently decreased IPSC amplitude. (j) Bar graph showing that cholinergic pairing increased SC stimulation evoked EPSP (**p = 0.002, n = 6 slices, t-test) and EPSC (***p < 0.001, n = 6 slices, t-test) amplitudes, while it decreased IPSC (**p = 0.004, n = 6 slices, t-test) amplitudes recorded in EC V/VI pyramidal neurons. (k,l) Paired-pulse (100-ms interval) responses showing that cholinergic pairing significantly decreased EPSC paired-pulse ratios (**p = 0.003, n = 6 slices, paired t-test), while it increased IPSC ratios in EC V/VI pyramidal neurons (**p = 0.008, n = 7 slices, paired t-test).
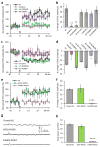
(a) Normalized SC-evoked EPSC responses from CA1 pyramidal neurons showing that the enhancement of hippocampal excitatory transmission by cholinergic pairing (at the time of 0 min as indicated by the arrow) can be blocked by either the non-selective muscarinic receptor antagonist atropine or by selective M1 receptor antagonist VU0255035, but not by any of the other receptor subtype selective antagonists. (b) Bar graph showing that only the non-selective muscarinic receptor antagonist atropine and the M1 receptor selective antagonist VU 0255035 significantly blocked cholinergic enhancement of hippocampal excitatory transmission; antagonists selective for M2, M3, M4 and M5 did not block cholinergic enhancement of EPSC amplitude. ** p = 0.0014 for atropine and 0.0013 for VU 0255035 compared with control, n = 6 slices per treatment group, One Way ANOVA, Holm-Sidak post hoc test. (c) Normalized SC-evoked IPSC amplitude from CA1 pyramidal neurons showing that cholinergic depression of hippocampal inhibitory transmission can be blocked by either the non-specific muscarinic receptor antagonist atropine or by M4 receptor selective antagonist PD102807, but not by any of the other selective antagonists. (d) Bar graph showing that besides non-specific muscarinic receptor antagonist (* p = 0.0121), M4 receptor selective antagonist PD 102807 (* p = 0.0138) was the only one that significantly blocked cholinergic depression of hippocampal inhibitory transmission. * compared with control group, n = 6 slices per treatment group, One Way ANOVA, Holm-Sidak post hoc test. (e, f) Normalized SC-evoked EPSC responses from CA1 pyramidal neurons (e) and bar graph (f) showing that cholinergic enhancement of hippocampal excitatory transmission can be blocked by knocking out M1 receptors in pyramidal neurons (CaMK2a-M1KO) but not in interneurons (GAD2-M1KO). **** p < 0.0001 compared with control slices from floxed M1 mice, n = 6 slices per group, One Way ANOVA, Holm-Sidak post hoc test. (g, h) Representative traces (g) and bar graph (h) showing that in vitro theta generation was impaired in slices with M1 receptor knockout in pyramidal neurons but not in slices with M1 receptor knockout in interneurons. **** p < 0.0001 compared with slices from floxed M1 controls, n = 6 slices per group, One Way ANOVA, Holm-Sidak post hoc test.
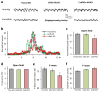
(a) Representative hippocampal local field potential recordings from mice with M1 mAChR knocked out from interneurons (GAD2-cre/floxed M1) or pyramidal neurons (CaMK2a-cre/floxed M1) or cre−/floxed M1 control mice. (b) Power spectral density analysis of representative recordings showing that the theta power was decreased in mice with M1 receptor knockout in pyramidal neurons but not M1 receptor knockout in interneurons. (c) Bar graph showing reduced peak theta power from mice with M1 knockout in pyramidal neurons (* p = 0.032) but not M1 knockout in interneurons (p = 0.793). * compared with floxed M1 controls, n = 7 mice per group, one-way ANOVA, Holm-Sidak post hoc test. (d) Bar graph showing that theta frequency was not significantly changed by M1 deletion. p = 0.7703 between groups, n = 7 mice per group, one-way ANOVA. (e) Bar graph showing that Y-maze spontaneous alternation task performance was impaired in mice with the M1 receptor knockout in pyramidal neurons (* p = 0.023) but not M1 receptor knockout in interneurons (p = 0.499). * compared with floxed M1 controls, n = 10 mice per group, one-way ANOVA, Holm-Sidak post hoc test. (f) Bar graph showing that the total number of Y-maze arm entries was not significantly changed among the three groups (p = 0.3889, n = 10 mice per group, one-way ANOVA).
Similar articles
-
Inducing theta oscillations in the entorhinal hippocampal network in vitro.
Gu Z, Yakel JL. Gu Z, et al. Brain Struct Funct. 2017 Mar;222(2):943-955. doi: 10.1007/s00429-016-1256-3. Epub 2016 Jul 1. Brain Struct Funct. 2017. PMID: 27369465 Free PMC article.
-
Gamma oscillations induced by kainate receptor activation in the entorhinal cortex in vitro.
Cunningham MO, Davies CH, Buhl EH, Kopell N, Whittington MA. Cunningham MO, et al. J Neurosci. 2003 Oct 29;23(30):9761-9. doi: 10.1523/JNEUROSCI.23-30-09761.2003. J Neurosci. 2003. PMID: 14586003 Free PMC article.
-
Theta oscillations in the hippocampus.
Buzsáki G. Buzsáki G. Neuron. 2002 Jan 31;33(3):325-40. doi: 10.1016/s0896-6273(02)00586-x. Neuron. 2002. PMID: 11832222 Review.
-
Smythe JW, Colom LV, Bland BH. Smythe JW, et al. Neurosci Biobehav Rev. 1992 Fall;16(3):289-308. doi: 10.1016/s0149-7634(05)80203-9. Neurosci Biobehav Rev. 1992. PMID: 1528522 Review.
Cited by
-
The Theta Rhythm of the Hippocampus: From Neuronal and Circuit Mechanisms to Behavior.
Nuñez A, Buño W. Nuñez A, et al. Front Cell Neurosci. 2021 Mar 4;15:649262. doi: 10.3389/fncel.2021.649262. eCollection 2021. Front Cell Neurosci. 2021. PMID: 33746716 Free PMC article.
-
Neural mechanisms of navigation involving interactions of cortical and subcortical structures.
Hinman JR, Dannenberg H, Alexander AS, Hasselmo ME. Hinman JR, et al. J Neurophysiol. 2018 Jun 1;119(6):2007-2029. doi: 10.1152/jn.00498.2017. Epub 2018 Feb 14. J Neurophysiol. 2018. PMID: 29442559 Free PMC article. Review.
-
A Model of the CA1 Field Rhythms.
Mysin I. Mysin I. eNeuro. 2021 Nov 8;8(6):ENEURO.0192-21.2021. doi: 10.1523/ENEURO.0192-21.2021. Print 2021 Nov-Dec. eNeuro. 2021. PMID: 34670820 Free PMC article.
-
Xiao Q, Lu M, Zhang X, Guan J, Li X, Wen R, Wang N, Qian L, Liao Y, Zhang Z, Liao X, Jiang C, Yue F, Ren S, Xia J, Hu J, Luo F, Hu Z, He C. Xiao Q, et al. Nat Commun. 2024 Oct 25;15(1):9231. doi: 10.1038/s41467-024-53522-9. Nat Commun. 2024. PMID: 39455583 Free PMC article.
-
Goral RO, Harper KM, Bernstein BJ, Fry SA, Lamb PW, Moy SS, Cushman JD, Yakel JL. Goral RO, et al. Front Behav Neurosci. 2022 Nov 24;16:1067409. doi: 10.3389/fnbeh.2022.1067409. eCollection 2022. Front Behav Neurosci. 2022. PMID: 36505727 Free PMC article.
References
-
- Battaglia FP, Benchenane K, Sirota A, Pennartz CM, Wiener SI. The hippocampus: hub of brain network communication for memory. Trends in cognitive sciences. 2011;15:310–318. - PubMed
-
- Buzsaki G. Theta oscillations in the hippocampus. Neuron. 2002;33:325–340. - PubMed
-
- Buzsaki G. Theta rhythm of navigation: link between path integration and landmark navigation, episodic and semantic memory. Hippocampus. 2005;15:827–840. - PubMed
MeSH terms
Substances
LinkOut - more resources
Full Text Sources
Other Literature Sources
Molecular Biology Databases
Research Materials