Comprehensive review of cardiovascular toxicity of drugs and related agents - PubMed
Review
. 2018 Jul;38(4):1332-1403.
doi: 10.1002/med.21476. Epub 2018 Jan 5.
Lenka Applová 1 , Jiří Patočka 2 3 , Vera Marisa Costa 4 , Fernando Remiao 4 , Jana Pourová 1 , Aleš Mladěnka 5 , Jana Karlíčková 6 , Luděk Jahodář 6 , Marie Vopršalová 1 , Kurt J Varner 7 , Martin Štěrba 8 ; TOX-OER and CARDIOTOX Hradec Králové Researchers and Collaborators
Affiliations
- PMID: 29315692
- PMCID: PMC6033155
- DOI: 10.1002/med.21476
Review
Comprehensive review of cardiovascular toxicity of drugs and related agents
Přemysl Mladěnka et al. Med Res Rev. 2018 Jul.
Abstract
Cardiovascular diseases are a leading cause of morbidity and mortality in most developed countries of the world. Pharmaceuticals, illicit drugs, and toxins can significantly contribute to the overall cardiovascular burden and thus deserve attention. The present article is a systematic overview of drugs that may induce distinct cardiovascular toxicity. The compounds are classified into agents that have significant effects on the heart, blood vessels, or both. The mechanism(s) of toxic action are discussed and treatment modalities are briefly mentioned in relevant cases. Due to the large number of clinically relevant compounds discussed, this article could be of interest to a broad audience including pharmacologists and toxicologists, pharmacists, physicians, and medicinal chemists. Particular emphasis is given to clinically relevant topics including the cardiovascular toxicity of illicit sympathomimetic drugs (e.g., cocaine, amphetamines, cathinones), drugs that prolong the QT interval, antidysrhythmic drugs, digoxin and other cardioactive steroids, beta-blockers, calcium channel blockers, female hormones, nonsteroidal anti-inflammatory, and anticancer compounds encompassing anthracyclines and novel targeted therapy interfering with the HER2 or the vascular endothelial growth factor pathway.
Keywords: dysrhythmia; heart failure; hypertension; myocardial infarction; stroke.
© 2018 The Authors Medicinal Research Reviews Published by Wiley Periodicals, Inc.
Figures
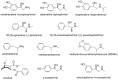
Endogenous catecholamines (noradrenaline and adrenaline) and clinically + toxicologically important sympathomimetics.
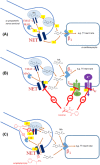
Noradrenaline release from sympathetic fibers at the synaptic cleft in the heart and the influence of indirect sympathomimetics. Physiological situation (A): upon stimulation of the sympathetic system, vesicles containing noradrenaline fuse with the cytoplasmic membrane of the synaptic cleft (1), noradrenaline (NA) is released (2), and stimulates β1‐adrenergic receptors (3). The effect of noradrenaline is, however, rapidly terminated (4) by the uptake (reuptake) mechanism via noradrenaline transporter (NET) and, thus, noradrenaline can be transported back to the vesicles (5) by vesicular monoamine transporter‐2 (VMAT‐2) or metabolized by MAO‐A (6, MAO). Effect of cocaine (B): cocaine blocks NET (7) and hence the half‐life and concentration of noradrenaline in the synaptic cleft is prolonged and increased, respectively. It also reduces the Na+ current (8, INa) and the rapid component of delayed rectifier current (9, I Kr). Effect of amphetamine (C): amphetamine replaces noradrenaline in vesicles (10) and blocks the uptake by VMAT‐2 (11) and metabolism by MAO‐A (12). This results in noradrenaline release into the cytosol. NET seems to work in the opposite direction (13) when the cytosolic concentration of noradrenaline is increased. On the other hand, NET is also partly blocked by amphetamine.
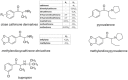
Chemical structure of cathinone derivatives “bath salts” and bupropion.
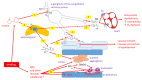
Simplified overview of the cardiovascular effect of cigarette smoke with separation of the effect of nicotine and other compounds generated by smoking. Nicotine stimulates nicotine receptors (NN) both in the sympathetic nervous system ganglia (1) and in the adrenal medulla (2). The former leads to release of noradrenaline (NA) from the sympathetic nerve terminals both in the heart (3) and vascular beds (4). The effect in the adrenal medulla leads mainly to the release of adrenaline (A) into the systemic blood circulation (5). The effect on the heart and majority of vessels is similar (6). In addition, adrenaline stimulates platelet aggregation (7). ROS (8) are formed and react with NO leading to the production of peroxynitrite (ONOO−), a highly toxic reactive species. Stimulation of platelet aggregation and activation of the immune system with subsequent inflammatory reaction is a consequence. ACh: acetylcholine.
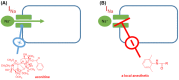
Drugs acting on Na+ channels. (A) Aconitine binds to the open state of the channel and blocks channel closing, therefore Na+ can cross the plasmatic membrane continuously, (B) an amide local anesthetic blocks channel opening and thus Na+ influx necessary for action potential generation and conduction.
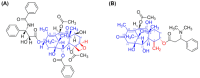
Chemical differences between taxanes and taxines. (A) Taxan taxol and (B) taxine B. Blue shows the basic core for the most common taxines and taxanes, while red highlights differences between taxanes and taxines.
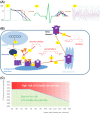
QT interval and torsade de pointes. (A) Different scenarios of QT prolongation. (1) Action potential. Normal action potential is shown in green, the prolongation of QT mainly due to plateau prolongation is shown in red, QT prolongations where rapid repolarization phase is also prolonged in black and blue (here the triangulation is apparent). (2) Corresponding QT prolongation on ECG. Marked QT prolongation, in particular with triangulation, might result in torsade de pointes (3). (B) Complicated synthesis and trafficking of hERG channels. After transcription to mRNA (1), primary the polypeptide is formed in ribosomes (2). In endoplasmic reticulum it is first associated with chaperons (3, heat shock proteins 70 and 90) and then four units are assembled into a tetramer forming the K+ channel (4). Thereafter, the immature channel is transported into the Golgi apparatus for final glycosylation (5). This mature protein is trafficked to the plasma membrane (6). Drugs can interfere with this process in different ways: arsenic trioxide hinders the formation of the chaperon–hERG polypeptide complex (7), pentamidine binds to the tetramer and blocks the transport from the endoplasmic reticulum (8), or ibutilide is a representative of many drugs that directly block the active channel (9). (C) QT interval nomogram according to Isbister270 and Isbister et al.286
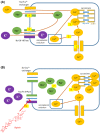
Calcium trafficking in cardiomyocytes under physiological conditions (A) and mechanism of action of digoxin (B). Ca2+ is transported into the cell mainly through voltage gated L‐type Ca2+ channels (1). An increase in intracellular Ca2+ levels triggers Ca2+ release from sarcoplasmic reticulum (2). Ca2+ is needed for interaction with actin‐myosin resulting in muscle contraction (3). Ca2+ is then returned back to sarcoplasmic reticulum (not shown) and to the extracellular space by the Na+/Ca2+ exchanger (4). By this transporter, Na+ is transported inside the cells and its level on both sides must be recovered by activity of sodium–potassium adenosine triphosphatase (Na+/K+‐ATPase, 5). Digoxin and other cardioactive steroids (B) block Na+/K+‐ATPase (6) and thus increase the intracellular Na+ levels (7). Higher intracellular Na+ levels block the passive and thus concentration‐dependent exchange of Ca2+ and Na+ through the Ca2+‐Na+ exchanger. This results in higher intracellular level of Ca2+ (8) available for myocardial contraction.
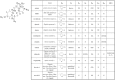
Chemical structures and sources of the major natural inhibitors of Na+/K+‐ATPase.
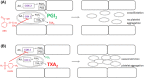
Different effects of low dose of acetylsalicylic acid and coxibs on the vascular system. (A) Acetylsalicylic acid (ASA) at low doses blocks mainly platelet cyclooxygenase 1, resulting in a relative excess of prostacyclin (PGI2) over thromboxane A2 (TxA2), vasodilation, and inhibition of platelet aggregation. (B) Coxibs are selective for cyclooxygenase 2. Therefore, their administration leads to excess of TxA2 with subsequent risk of vasoconstriction and platelet aggregation.
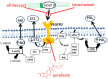
Examples of drugs acting on the VEGF pathway. This figure shows drugs that can inhibit the VEGF pathway and hence decrease the production of NO and prostacyclin (PGI2). The role of endothelin 1 (ET‐1) is less known. Alflibercept and bevacizumab bind directly to vascular endothelial growth factor A (VEGF A), while small drugs such as sunitinib block the tyrosine kinase domain (TK) of the vascular endothelial growth factor receptor 2 (VEGFR2). AA: arachidonic acid; Akt: protein kinase B (serine/threonine‐specific protein kinase); cPLA: cytosolic phospholipase A2; DAG: diacylglycerol; ERG: transcription factor; eNOS: endothelial NO‐synthase; MEK: mitogen‐activated protein kinase kinase; P‐eNOS: phosphorylated eNOS; PI3K: phosphoinositide 3‐kinase; PKC: protein kinase C; PLAγ: phospholipase γ1 (also known as phospholipase C); Raf: a serine/threonine‐specific protein kinase.
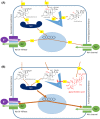
Aldosterone/cortisol activity in a mineralocorticoid sensitive kidney tubule cell under physiological conditions (A), or after inhibition by glycyrrhetinic acid (B). (1) Both aldosterone and cortisol are lipophilic enough to enter the cells, (2) cortisol is, however, immediately metabolized by the enzyme 11‐β‐hydroxysteroid dehydrogenase type 2 (E) into cortisone, which does not have affinity for the mineralocorticoid receptor (MR), (3) binding of aldosterone to MR begins the cascade. Through DNA binding (4), the expression of Na+ channels and Na+/K+‐ATPase (5) is upregulated. When glycyrrhetinic acid is present in plasma, it can penetrate into cells (6), where it has a high affinity for the enzyme (7). As a consequence of enzyme inhibition, cortisol is not inactivated and binds to MR (8) and the same cascade (part A, 4–5) occurs without the need for aldosterone.
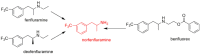
Norfenfluramine, the common metabolite of fenfluramine and benfluorex.
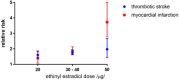
Relative risk of stroke and myocardial infarction in relation to the dose of estrogen (ethinyl estradiol). The term thrombotic stroke means cerebral infarction due to cerebral thrombosis or embolism. Figure shows the relative risk with 95% confidence intervals. The figure was prepared using data from the clinical study of Lidegaard et al.494
Similar articles
-
Bleumink GS, Feenstra J, Stricker BH. Bleumink GS, et al. Ned Tijdschr Geneeskd. 2001 Jun 23;145(25):1200-3. Ned Tijdschr Geneeskd. 2001. PMID: 11447875 Review. Dutch.
-
Negrusz-Kawecka M. Negrusz-Kawecka M. Pol Merkur Lekarski. 2001 Sep;11(63):271-5. Pol Merkur Lekarski. 2001. PMID: 11761828 Review. Polish.
-
Pettirossi G, Paoletti V, Mammarella A, Fioravanti S, Scodanibbio MC. Pettirossi G, et al. Clin Ter. 1996 Jan-Feb;147(1-2):67-77. Clin Ter. 1996. PMID: 8767957 Review. Italian.
-
Treatment of cocaine cardiovascular toxicity: a systematic review.
Richards JR, Garber D, Laurin EG, Albertson TE, Derlet RW, Amsterdam EA, Olson KR, Ramoska EA, Lange RA. Richards JR, et al. Clin Toxicol (Phila). 2016 Jun;54(5):345-64. doi: 10.3109/15563650.2016.1142090. Epub 2016 Feb 26. Clin Toxicol (Phila). 2016. PMID: 26919414 Review.
-
First-line diuretics versus other classes of antihypertensive drugs for hypertension.
Reinhart M, Puil L, Salzwedel DM, Wright JM. Reinhart M, et al. Cochrane Database Syst Rev. 2023 Jul 13;7(7):CD008161. doi: 10.1002/14651858.CD008161.pub3. Cochrane Database Syst Rev. 2023. PMID: 37439548 Free PMC article. Review.
Cited by
-
Ramadan M, Cooper B, Posnack NG. Ramadan M, et al. Birth Defects Res. 2020 Oct;112(17):1362-1385. doi: 10.1002/bdr2.1752. Epub 2020 Jul 21. Birth Defects Res. 2020. PMID: 32691967 Free PMC article. Review.
-
Kadioglu O, Klauck SM, Fleischer E, Shan L, Efferth T. Kadioglu O, et al. Arch Toxicol. 2021 Jul;95(7):2485-2495. doi: 10.1007/s00204-021-03058-4. Epub 2021 May 22. Arch Toxicol. 2021. PMID: 34021777 Free PMC article.
-
Narkar A, Willard JM, Blinova K. Narkar A, et al. Int J Mol Sci. 2022 Mar 16;23(6):3199. doi: 10.3390/ijms23063199. Int J Mol Sci. 2022. PMID: 35328619 Free PMC article. Review.
-
Can Isoquinoline Alkaloids Affect Platelet Aggregation in Whole Human Blood?
Parvin MS, Hrubša M, Fadraersada J, Carazo A, Karlíčková J, Cahlíková L, Chlebek J, Macáková K, Mladěnka P. Parvin MS, et al. Toxins (Basel). 2022 Jul 15;14(7):491. doi: 10.3390/toxins14070491. Toxins (Basel). 2022. PMID: 35878229 Free PMC article.
-
Matsukawa A, Yanagisawa T, Parizi MK, Laukhtina E, Klemm J, Fazekas T, Mori K, Kimura S, Briganti A, Ploussard G, Karakiewicz PI, Miki J, Kimura T, Rajwa P, Shariat SF. Matsukawa A, et al. Prostate Cancer Prostatic Dis. 2024 Sep 5. doi: 10.1038/s41391-024-00886-0. Online ahead of print. Prostate Cancer Prostatic Dis. 2024. PMID: 39237679 Review.
References
-
- Klaassen CD. Casarett and Doull's Toxicology: The Basic Science of Poisons. 7th ed New York: McGraw‐Hill; 2008.
-
- Reichel F‐X, Ritter L. Illustrated Handbook of Toxicology. 4th ed Stuttgart: Thieme; 2011.
-
- Olson KR, Anderson, IB , Benowitz NL, Poisoning & Drug Overdose. 5th ed New York: McGraw‐Hill; 2006.
-
- Shannon MW, Borron SW, Burns M. Haddad and Winchester´s Clinical Management of Poisoning and Drug Overdose. 4th ed Philadelphia: Sunders/Elsevier; 2007.
-
- Bryson PD. Comprehensive Review in Toxicology for Emergency Clinicians. 3rd ed Washington: Taylor & Francis; 1997.
Publication types
MeSH terms
Substances
LinkOut - more resources
Full Text Sources
Other Literature Sources
Research Materials
Miscellaneous