Ocean convergence and the dispersion of flotsam - PubMed
- ️Mon Jan 01 2018
. 2018 Feb 6;115(6):1162-1167.
doi: 10.1073/pnas.1718453115. Epub 2018 Jan 16.
Andrey Y Shcherbina 2 , Jody M Klymak 3 4 , Jeroen Molemaker 5 , Guillaume Novelli 6 , Cédric M Guigand 6 , Angelique C Haza 6 , Brian K Haus 6 , Edward H Ryan 6 , Gregg A Jacobs 7 , Helga S Huntley 8 , Nathan J M Laxague 9 , Shuyi Chen 10 , Falko Judt 11 , James C McWilliams 5 , Roy Barkan 5 , A D Kirwan Jr 8 , Andrew C Poje 12 , Tamay M Özgökmen 6
Affiliations
- PMID: 29339497
- PMCID: PMC5819445
- DOI: 10.1073/pnas.1718453115
Ocean convergence and the dispersion of flotsam
Eric A D'Asaro et al. Proc Natl Acad Sci U S A. 2018.
Erratum in
-
Correction for D'Asaro et al., Ocean convergence and the dispersion of flotsam.
[No authors listed] [No authors listed] Proc Natl Acad Sci U S A. 2018 Mar 13;115(11):E2664. doi: 10.1073/pnas.1802701115. Epub 2018 Mar 5. Proc Natl Acad Sci U S A. 2018. PMID: 29507188 Free PMC article. No abstract available.
Abstract
Floating oil, plastics, and marine organisms are continually redistributed by ocean surface currents. Prediction of their resulting distribution on the surface is a fundamental, long-standing, and practically important problem. The dominant paradigm is dispersion within the dynamical context of a nondivergent flow: objects initially close together will on average spread apart but the area of surface patches of material does not change. Although this paradigm is likely valid at mesoscales, larger than 100 km in horizontal scale, recent theoretical studies of submesoscales (less than ∼10 km) predict strong surface convergences and downwelling associated with horizontal density fronts and cyclonic vortices. Here we show that such structures can dramatically concentrate floating material. More than half of an array of ∼200 surface drifters covering ∼20 × 20 km2 converged into a 60 × 60 m region within a week, a factor of more than 105 decrease in area, before slowly dispersing. As predicted, the convergence occurred at density fronts and with cyclonic vorticity. A zipperlike structure may play an important role. Cyclonic vorticity and vertical velocity reached 0.001 s-1 and 0.01 ms-1, respectively, which is much larger than usually inferred. This suggests a paradigm in which nearby objects form submesoscale clusters, and these clusters then spread apart. Together, these effects set both the overall extent and the finescale texture of a patch of floating material. Material concentrated at submesoscale convergences can create unique communities of organisms, amplify impacts of toxic material, and create opportunities to more efficiently recover such material.
Keywords: dispersion; eddy; ocean; submesoscale; vertical velocity.
Copyright © 2018 the Author(s). Published by PNAS.
Conflict of interest statement
Conflict of interest statement: E.A.D. and T.F. are coauthors on a 2014 paper. This was a brief announcement that did not involve any scientific collaboration.
Figures
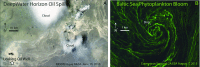
Distribution of floating materials from satellite images. (A) Oil from Deepwater Horizon spill seen in a sunglint image. (B) Cyanobacteria bloom in the central Baltic Sea (57.7°N, 20.7°E, water depth 135 m). Dark line is the wake of a ship.
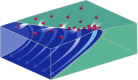
Ocean surface currents converge and sink at a density front separating light and heavy water, sweeping floating material to the front where it accumulates.
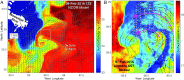
Experiment location and environment. (A) Surface density (colors) in the experimental region as simulated by the Navy Coastal Ocean Model (NCOM) (
SI Materials and Methods). Surface velocity (vectors) and bottom depth (gray lines) are shown. (B) Aircraft survey of SST. Location is shown by the white box in A. Although not an accurate measure of surface density, SST captures the general eddy structure (
Fig. S4). Initial drifter positions (white dots), vectors of 7 m velocity measured by the ship survey and streamfunction (black lines) computed (
SI Materials and Methods) from these velocities are shown. Magenta arrows show the sense of circulation.
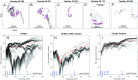
Evolution of the drifter array. (A–F) Drifter positions at selected times, a subset of the online animation (
SI Animation of Drifter Evolution). (E, Inset) Detail of a 60-m-wide cluster of 127 drifters, colored magenta, at its smallest. Each panel is centered on these magenta drifters; nearly all of the other drifters exit the frame by F. Gray lines show 7,500-s-long “tails” of drifter motion. (G) Distribution of drifter pair separations as a function of time (gray shading indicates the number of pairs in each of the 100 logarithmically spaced bins; larger numbers are darker). RMS pair separation (red) and times of A–F (blue lines) are shown. (H) Same, but for separations of drifters from the center of drifter clusters (
SI Drifter Cluster Analysis). (I) Same, but for separations between the centers of the drifter clusters. Grayscale has been adjusted to compensate for the different numbers of pairs in different panels.
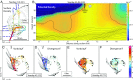
A convergent submesoscale structure. (A) Surface density (colored dots) from ship sections across the drifter array and drifter trajectories (gray) during the same time period (black dot marks trajectory end). Positions are plotted in a coordinate system moving with the cyclonic eddy (eastward at 0.13 m s−1, southward at 0.05 m s−1). A section is highlighted in red. (B) Potential density (colors and contours) along the highlighted section. Data are averaged over 3 m vertically and 1 km horizontally. Red dots indicate surface positions of individual profiles. Large gray circle, duplicated in other panels, marks the location of densest surface water on yearday ∼42.5. (C) Vorticity/f (colored ellipses) computed (
SI Estimating Vorticity and Divergence from Drifter Data) from drifters on yearday 42.522 and drifter trajectories during the previous 5,000 s (gray line ending at black dot). Ellipse shape shows the spatial distribution of drifters; only data with ellipses with a major to minor axis ratio less than 5 are used. (D) Divergence/f at the same time. (E and F) Same as C and D but at yearday 43.772.
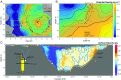
Sinking of water at the front. (A) Surface density colored and contoured using same color map as in Fig. 5. Thin lines show ship survey lines; yellow lines indicate the section plotted in B; circles show stations. Mapping uses objective analysis with a 1.2-km Gaussian correlation function. Black dots show drifter positions with 5,200-s tails, the duration of the survey. (B) Section of potential density using all data along yellow tracks (
SI Float Navigation). Small white dots show profile locations. Data are smoothed with 2 m vertical and 500 m horizontal scales. (C) Sketch of the Lagrangian float and vertical velocity (colors) computed from its data. Float was deployed at the location of the top star in A and recovered at the bottom star. Estimated trajectory (
SI Float Navigation) is shown by the red lines in A and B and marked by white symbols common to all panels. Solid lines are more certain than dashed ones. Horizontal arrows in B indicate uncertainty in the cross-frontal position. Vertical velocity in C is computed from the float’s vertical motion (colored line) and from the upward-looking ADCP (colors and contours). Long arrows in each panel indicate the sense of circulation.
Similar articles
-
Yu X, Barkan R, Naveira Garabato AC. Yu X, et al. Nat Commun. 2024 Oct 25;15(1):9214. doi: 10.1038/s41467-024-53551-4. Nat Commun. 2024. PMID: 39455553 Free PMC article.
-
Submesoscale currents in the ocean.
McWilliams JC. McWilliams JC. Proc Math Phys Eng Sci. 2016 May;472(2189):20160117. doi: 10.1098/rspa.2016.0117. Proc Math Phys Eng Sci. 2016. PMID: 27279778 Free PMC article. Review.
-
Submesoscale Rossby waves on the Antarctic circumpolar current.
Taylor JR, Bachman S, Stamper M, Hosegood P, Adams K, Sallee JB, Torres R. Taylor JR, et al. Sci Adv. 2018 Mar 28;4(3):eaao2824. doi: 10.1126/sciadv.aao2824. eCollection 2018 Mar. Sci Adv. 2018. PMID: 29670936 Free PMC article.
-
Ocean submesoscales as a key component of the global heat budget.
Su Z, Wang J, Klein P, Thompson AF, Menemenlis D. Su Z, et al. Nat Commun. 2018 Feb 22;9(1):775. doi: 10.1038/s41467-018-02983-w. Nat Commun. 2018. PMID: 29472586 Free PMC article.
-
The Impact of Submesoscale Physics on Primary Productivity of Plankton.
Mahadevan A. Mahadevan A. Ann Rev Mar Sci. 2016;8:161-84. doi: 10.1146/annurev-marine-010814-015912. Epub 2015 Sep 21. Ann Rev Mar Sci. 2016. PMID: 26394203 Review.
Cited by
-
News Feature: The perplexing physics of oil dispersants.
Waldrop MM. Waldrop MM. Proc Natl Acad Sci U S A. 2019 May 28;116(22):10603-10607. doi: 10.1073/pnas.1907155116. Proc Natl Acad Sci U S A. 2019. PMID: 31138709 Free PMC article. No abstract available.
-
Proof of concept for a new sensor to monitor marine litter from space.
Cózar A, Arias M, Suaria G, Viejo J, Aliani S, Koutroulis A, Delaney J, Bonnery G, Macías D, de Vries R, Sumerot R, Morales-Caselles C, Turiel A, González-Fernández D, Corradi P. Cózar A, et al. Nat Commun. 2024 Jun 14;15(1):4637. doi: 10.1038/s41467-024-48674-7. Nat Commun. 2024. PMID: 38877039 Free PMC article.
-
Yu X, Barkan R, Naveira Garabato AC. Yu X, et al. Nat Commun. 2024 Oct 25;15(1):9214. doi: 10.1038/s41467-024-53551-4. Nat Commun. 2024. PMID: 39455553 Free PMC article.
-
Direct observational evidence of an oceanic dual kinetic energy cascade and its seasonality.
Balwada D, Xie JH, Marino R, Feraco F. Balwada D, et al. Sci Adv. 2022 Oct 14;8(41):eabq2566. doi: 10.1126/sciadv.abq2566. Epub 2022 Oct 12. Sci Adv. 2022. PMID: 36223461 Free PMC article.
-
Milke F, Meyerjürgens J, Simon M. Milke F, et al. Nat Commun. 2023 Oct 2;14(1):6141. doi: 10.1038/s41467-023-41909-z. Nat Commun. 2023. PMID: 37783696 Free PMC article.
References
-
- Munk W, Armi L, Fischer K, Zachariasen F. Spirals on the sea. Proc R Soc A. 2000;456:1217–1280.
-
- Fedorov KN, Ginsburg AI. 1992. The Near-Surface Layer of the Ocean, trans Rosenberg M (VSP, Utrecht, Netherlands)
-
- Flament P, Armi L. The shear, convergence, and thermohaline structure of a front. J Phys Oceanogr. 2000;30:51–66.
-
- Zhon Y, Bracco A, Villareal TA. Pattern formation at the ocean surface: Sargassum distribution and the role of the eddy field. Limnol Oceanogr. 2012;2:12–27.
-
- Richardson LF. Atmospheric diffusion shown on a distance-neighbor graph. Proc R Soc A. 1926;110:709–737.
Publication types
LinkOut - more resources
Full Text Sources
Other Literature Sources
Research Materials