A calcium-sensing receptor mutation causing hypocalcemia disrupts a transmembrane salt bridge to activate β-arrestin-biased signaling - PubMed
- ️Mon Jan 01 2018
A calcium-sensing receptor mutation causing hypocalcemia disrupts a transmembrane salt bridge to activate β-arrestin-biased signaling
Caroline M Gorvin et al. Sci Signal. 2018.
Abstract
The calcium-sensing receptor (CaSR) is a G protein-coupled receptor (GPCR) that signals through Gq/11 and Gi/o to stimulate cytosolic calcium (Ca2+i) and mitogen-activated protein kinase (MAPK) signaling to control extracellular calcium homeostasis. Studies of loss- and gain-of-function CASR mutations, which cause familial hypocalciuric hypercalcemia type 1 (FHH1) and autosomal dominant hypocalcemia type 1 (ADH1), respectively, have revealed that the CaSR signals in a biased manner. Thus, some mutations associated with FHH1 lead to signaling predominantly through the MAPK pathway, whereas mutations associated with ADH1 preferentially enhance Ca2+i responses. We report a previously unidentified ADH1-associated R680G CaSR mutation, which led to the identification of a CaSR structural motif that mediates biased signaling. Expressing CaSRR680G in HEK 293 cells showed that this mutation increased MAPK signaling without altering Ca2+i responses. Moreover, this gain of function in MAPK activity occurred independently of Gq/11 and Gi/o and was mediated instead by a noncanonical pathway involving β-arrestin proteins. Homology modeling and mutagenesis studies showed that the R680G CaSR mutation selectively enhanced β-arrestin signaling by disrupting a salt bridge formed between Arg680 and Glu767, which are located in CaSR transmembrane domain 3 and extracellular loop 2, respectively. Thus, our results demonstrate CaSR signaling through β-arrestin and the importance of the Arg680-Glu767 salt bridge in mediating signaling bias.
Copyright © 2018 The Authors, some rights reserved; exclusive licensee American Association for the Advancement of Science. No claim to original U.S. Government Works.
Conflict of interest statement
Competing interests: The authors declare that they have no competing interests.
Figures
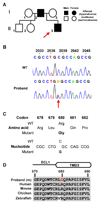
(A) Pedigree of family with ADH1. The proband (individual II.3) is indicated by an arrow. (B) A heterozygous C-to-G transition at nucleotide c.2038 was identified in the proband and his father by Sanger DNA sequencing and confirmed to cosegregate with hypocalcemia. (C) This C-to-G transition changes a CGC codon to GGC and is predicted to result in a missense amino acid substitution from Arg to Gly at position 680 in the CaSR protein. (D) Multiple sequence alignment of residues surrounding the Arg680 (R) residue encompassing extracellular loop 1 (ECL1) and transmembrane domain 3 (TMD3). The Arg680 (R) residue, which is evolutionarily conserved, is located within TMD3, and the mutant Gly680 (G) residue is shown in red. Conserved residues are shaded in gray.
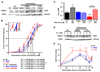
(A) Western blot analysis of HEK293 cells expressing wild-type (WT) or ADH1-associated mutant (R680G and L173F) CaSRs. Calnexin is a loading control. (B) Intracellular Ca2+ (Ca2+i) responses to changes in extracellular Ca2+ concentration ([Ca2+]e) in cells expressing the indicated wild-type or mutant CaSRs in the absence or presence of the allosteric CaSR inhibitor NPS-2143. Inset shows magnification of the curves between 2-3.5mM [Ca2+]e. Data are shown as the mean±SEM from 4-7 transfections, and EC50 values with 95% confidence intervals (CIs) are provided (F-test). (C) Histogram showing EC50 values with 95% CIs for cells expressing wild-type or R680G or L173F mutant CaSRs in the absence or presence of NPS-2143. (D) Western blot analysis of cells expressing the indicated wild-type and mutant forms of CaSR and used for assessment of NFAT reporter responses. (E) [Ca2+]e-induced NFAT reporter responses of cells expressing wild-type or mutant CaSRs. Responses at each [Ca2+]e are shown as a fold-change of basal (0.1mM) [Ca2+]e responses and presented as mean±SEM of 4 transfections. *p<0.05, **p<0.01 ****p<0.0001 using 2-way ANOVA with Tukey’s multiple-comparisons tests versus cells expressing wild-type CaSR at each [Ca2+]e.
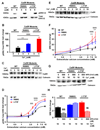
(A) Western blot analysis showing Ca2+e-induced phosphorylation of ERK1/2 (pERK1/2) in HEK293 cells expressing wild-type (WT) or ADH1-associated CaSR mutants (R680G or L173F). (B) Densitometric analysis of Western blot data in panel A. (C) Western blot analysis showing transgenic expression of the indicated forms of CaSR in cells used to assess Ca2+e-induced phosphorylation of ERK1/2 by AlphaScreen analysis. Calnexin is a loading control. (D) Ca2+e-induced ERK1/2 phosphorylation in CaSR-expressing cells as measured by AlphaScreen analysis, shown as the ratio of phosphorylated ERK1/2 (pERK) to total ERK. (E) Western blot analysis showing transgenic expression of the indicated forms of CaSR in cells used to assess Ca2+e-induced SRE reporter activity. (F) Ca2+e-induced SRE reporter activity in CaSR-expressing cells. (G) Western blot analysis showing transgenic expression of CaSR in cells used to assess the effects of NPS-2143 on Ca2+e-induced SRE responses. (H) SRE reporter activity in CaSR-expressing cells in the absence or presence of the allosteric CaSR inhibitor. Data shows mean±SEM values for N=4-20 independent transfections. **p<0.01, ***p<0.001, ****p<0.0001 for CaSRR680G versus CaSRWT; $$$p<0.001, $$$$p<0.0001 for CaSRL173F versus CaSRWT in panels B, D and F. §§p<0.01, §§§p<0.001 for NPS-2143-treated cells compared to respective untreated cells in panel H. Two-way ANOVA with Tukey’s multiple-comparisons test.
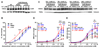
(A) Western blot analysis of HEK293 cells expressing wild-type (WT) or ADH1-associated CaSR mutants (R680G or L173F). These cells were used for assessment of IP1 responses. Calnexin is a loading control. (B) Ca2+e-induced IP1 fold change in cells expressing the indicated forms of CaSR. (C) Western blot analysis of cells used to assess the effect of the Gαq/11 inhibitors YM-254890 (YM) and UBO-QIC (UBO), on SRE reporter activity. (D) [Ca2+]e-induced SRE reporter activity in cells expressing the indicated forms of CaSR in the presence or absence of YM. (E) [Ca2+]e-induced SRE reporter in cells expressing the indicated forms of CaSR in the presence or absence of UBO-QIC. Data shows mean±SEM for 8-12 independent transfections. *p<0.05, **p<0.01 ****p<0.0001 for untreated cells expressing CaSRWT versus untreated (black) or YM- or UBO-QIC–treated (blue) cells expressing CaSRR690G in panels D and E. $p<0.05, $$p<0.01, $$$$p<0.0001 for untreated cells expressing CaSRWT versus untreated (black), or YM- or UBO-QIC–treated (red) cells expressing CaSRL173F in panels B, D and E (2-way ANOVA with Tukey’s multiple-comparisons test).
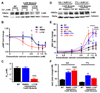
(A) Western blot analysis of HEK293 cells expressing wild-type (WT) or ADH1-associated CaSR mutants (R680G or L173F). These cells were used for assessment of cAMP responses. Calnexin is a loading control. (B) Ca2+e-induced fold change in cAMP abundance in cells expressing the indicated forms of CaSR. (C) Histograms showing the cAMP IC50 with 95% confidence intervals for cells expressing the indicated forms of CaSR. (D) Western blot analysis of cells expressing the indicated forms of CaSR in the presence of pertussis toxin (PTx) or vehicle (Veh). These cells were used to assess effect of PTx on SRE reporter activity. (E) Fold change in [Ca2+]e-induced SRE reporter activity in cells expressing the indicated forms of CaSR in the absence or presence of PTx. (F) Histograms showing area under the curve (AUC) of SRE reporter responses in vehicle- or PTx-treated cells expressing the indicated forms of CaSR. Data shows mean±SEM for 4-12 independent transfections. *p<0.05, ****p<0.0001 for CaSRR680G compared to CaSRWT in panels E-F. $p<0.05, $$$$p<0.0001 for CaSRL173F compared to CaSRWT in panels B, C, E and F (2-way ANOVA with Tukey’s multiple-comparisons test).
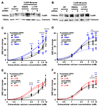
(A-B) Western blot analysis of HEK293 cells expressing wild-type (WT) or ADH1-associated CaSR mutants (R680G or L173F) and treated with scrambled siRNA (-) or siRNAs targeting (A) β-arrestin1 (βarr1) or (B) β-arrestin2 (βarr2). Calnexin was used as a loading control. (C-D) [Ca2+]e-induced SRE reporter responses in cells expressing CaSRR680G and treated with a scrambled siRNA or with siRNAs targeting (C) βarr1or (D) βarr2 or a scrambled siRNA. (E-F) [Ca2+]e-induced SRE reporter responses in cells expressing CaSRL173F and treated with siRNAs targeting βarr1 (E) or βarr2 (F) or a scrambled siRNA. The responses of cells treated with siRNAs targeting β-arrestin were compared to the respective cells treated with scrambled siRNA using a 2-way ANOVA with Tukey’s multiple-comparisons test. $p<0.05 and $$p<0.0001 for scrambled vs siRNA for cells expressing wild-type (black) or R680G (blue) CaSR; *p<0.05, **p<0.01, ***p<0.001, ***p<0.0001 for scrambled siRNA treatment of cells expressing wild-type CaSR vs mutant CaSR (blue) and scrambled siRNA treatment of cells expressing wild-type CaSR vs targeted (β-arrestin) siRNA treated mutant CaSR (black). Data is shown as mean±SEM for 8-16 independent transfections.
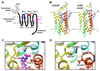
(A) Schematic diagram of a CaSR monomer showing the extracellular bi-lobed venus flytrap domain (VFTD), seven transmembrane domains (TMDs 1-7) with extracellular loops 1–3 (ECL1–3) and intracellular loops 1–3 (ICL1–3), and the cytoplasmic domain. The locations of Arg680 in TMD3 (R680, blue), Glu767 in ECL2 (E767, red), and Glu837 in TMD7 (E837, magenta) are indicated. (B) Ribbon diagram showing the transmembrane domains of mGluR1 derived from the published crystal structure (44) and a model of the CaSR transmembrane regions based on homology to mGluR1. TMDs 1-7 are numbered; e and i indicate the extracellular and cytoplasmic components of the plasma membrane, respectively. (C) Close-up view of the mGluR1 binding pocket for the negative allosteric modulator 4-fluoro-N-(4-(6-isopropylamino)pyrimidin-4-yl)thiazol-2-yl)-N-methylbenzamide (FITM) (magenta and purple molecule), and (D) the corresponding region in the CaSR. Distances between selected atoms are indicated with dashed lines.
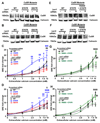
(A-B) Western blot analysis of HEK293 cells expressing wild-type or engineered mutant forms of CaSR (E767R or E837R) and treated with siRNAs targeting (A) β-arrestin1 (βarr1) or (B) β-arrestin2 (βarr2). (C-D) [Ca2+]e-induced SRE reporter responses in cells expressing CaSRE767R or CaSRE837R and treated with a scrambled siRNA or with siRNAs targeting (C) βarr1or (D) βarr2. (E-F) Western blot analysis of HEK293 cells expressing wild-type CaSR, E767R CaSR, or a double mutant (dm) form of CaSR incorporating both the R680E and E767R mutations and treated with a scrambled siRNA or siRNA targeting (E) βarr1 or (F) βarr2. These cells were used to assess SRE reporter activity following knockdown of βarr1 or βarr2. (G-H) [Ca2+]e-induced SRE reporter responses in cells expressing the double mutant CaSRR680E-E767R and treated with a scrambled siRNA or with siRNAs targeting (G) βarr1or (H) βarr2. Data shows mean±SEM for 8-16 independent transfections. *p<0.05, **p<0.001, ****p<0.0001 for E767R versus WT CaSR in panels C-D; $p<0.05, $$p<0.01, $$$$p<0.0001 for targeted versus scrambled siRNAs for wild-type (black) or E767R (blue) CaSR in panels C-D; $$p<0.01, $$$p<0.001, $$$$p<0.0001 for a comparison between Glu680-Arg767 or wild-type CaSR-expressing cells treated with targeted siRNA and respective cells treated with scrambled siRNA in panels G-H (2-way ANOVA with Tukey’s multiple-comparisons).
Similar articles
-
Dong B, Endo I, Ohnishi Y, Kondo T, Hasegawa T, Amizuka N, Kiyonari H, Shioi G, Abe M, Fukumoto S, Matsumoto T. Dong B, et al. J Bone Miner Res. 2015 Nov;30(11):1980-93. doi: 10.1002/jbmr.2551. Epub 2015 Jul 16. J Bone Miner Res. 2015. PMID: 25967373
-
Gorvin CM, Frost M, Malinauskas T, Cranston T, Boon H, Siebold C, Jones EY, Hannan FM, Thakker RV. Gorvin CM, et al. Hum Mol Genet. 2018 Nov 1;27(21):3720-3733. doi: 10.1093/hmg/ddy263. Hum Mol Genet. 2018. PMID: 30052933 Free PMC article.
-
Gorvin CM, Stokes VJ, Boon H, Cranston T, Glück AK, Bahl S, Homfray T, Aung T, Shine B, Lines KE, Hannan FM, Thakker RV. Gorvin CM, et al. J Clin Endocrinol Metab. 2020 Mar 1;105(3):952-63. doi: 10.1210/clinem/dgz251. J Clin Endocrinol Metab. 2020. PMID: 31820785 Free PMC article.
-
Gorvin CM. Gorvin CM. J Mol Endocrinol. 2018 Jul;61(1):R1-R12. doi: 10.1530/JME-18-0049. Epub 2018 Mar 29. J Mol Endocrinol. 2018. PMID: 29599414 Review.
-
Autosomal dominant hypocalcemia with a novel CASR mutation: a case study and literature review.
Wu Y, Zhang C, Huang X, Cao L, Liu S, Zhong P. Wu Y, et al. J Int Med Res. 2022 Jul;50(7):3000605221110489. doi: 10.1177/03000605221110489. J Int Med Res. 2022. PMID: 35818129 Free PMC article. Review.
Cited by
-
The calcium-sensing receptor in physiology and in calcitropic and noncalcitropic diseases.
Hannan FM, Kallay E, Chang W, Brandi ML, Thakker RV. Hannan FM, et al. Nat Rev Endocrinol. 2018 Dec;15(1):33-51. doi: 10.1038/s41574-018-0115-0. Nat Rev Endocrinol. 2018. PMID: 30443043 Free PMC article. Review.
-
Heterogeneity of G protein activation by the calcium-sensing receptor.
Abid HA, Inoue A, Gorvin CM. Abid HA, et al. J Mol Endocrinol. 2021 Jun 21;67(2):41-53. doi: 10.1530/JME-21-0058. J Mol Endocrinol. 2021. PMID: 34077389 Free PMC article.
-
Calcium-sensing receptor signaling - How human disease informs biology.
Gorvin CM. Gorvin CM. Curr Opin Endocr Metab Res. 2021 Feb;16:10-28. doi: 10.1016/j.coemr.2020.06.007. Epub 2020 Jul 2. Curr Opin Endocr Metab Res. 2021. PMID: 34141952 Free PMC article.
-
Werner LE, Wagner U. Werner LE, et al. Front Physiol. 2023 Jan 6;13:1078569. doi: 10.3389/fphys.2022.1078569. eCollection 2022. Front Physiol. 2023. PMID: 36685206 Free PMC article. Review.
-
Biased Signaling and Allosteric Modulation at the FSHR.
Landomiel F, De Pascali F, Raynaud P, Jean-Alphonse F, Yvinec R, Pellissier LP, Bozon V, Bruneau G, Crépieux P, Poupon A, Reiter E. Landomiel F, et al. Front Endocrinol (Lausanne). 2019 Mar 13;10:148. doi: 10.3389/fendo.2019.00148. eCollection 2019. Front Endocrinol (Lausanne). 2019. PMID: 30930853 Free PMC article. Review.
References
-
- Brown EM. Role of the calcium-sensing receptor in extracellular calcium homeostasis. Best practice & research. Clinical endocrinology & metabolism. 2013;27:333–343. - PubMed
-
- Chang W, Chen TH, Pratt S, Shoback D. Amino acids in the second and third intracellular loops of the parathyroid Ca2+-sensing receptor mediate efficient coupling to phospholipase C. The Journal of biological chemistry. 2000;275:19955–19963. - PubMed
-
- Goolam MA, Ward JH, Avlani VA, Leach K, Christopoulos A, Conigrave AD. Roles of intraloops-2 and -3 and the proximal C-terminus in signalling pathway selection from the human calcium-sensing receptor. FEBS letters. 2014;588:3340–3346. - PubMed
-
- Hofer AM, Brown EM. Extracellular calcium sensing and signalling. Nature reviews Molecular cell biology. 2003;4:530–538. - PubMed
-
- Kifor O, MacLeod RJ, Diaz R, Bai M, Yamaguchi T, Yao T, Kifor I, Brown EM. Regulation of MAP kinase by calcium-sensing receptor in bovine parathyroid and CaR-transfected HEK293 cells. Am J Physiol Renal Physiol. 2001;280:F291–302. - PubMed
Publication types
MeSH terms
Substances
Supplementary concepts
Grants and funding
- UC2 HL103010/HL/NHLBI NIH HHS/United States
- RC2 HL102926/HL/NHLBI NIH HHS/United States
- 106995/WT_/Wellcome Trust/United Kingdom
- RC2 HL102924/HL/NHLBI NIH HHS/United States
- 14414/CRUK_/Cancer Research UK/United Kingdom
- RC2 HL102923/HL/NHLBI NIH HHS/United States
- UC2 HL102926/HL/NHLBI NIH HHS/United States
- G1000467/MRC_/Medical Research Council/United Kingdom
- 26752/CRUK_/Cancer Research UK/United Kingdom
- UC2 HL102923/HL/NHLBI NIH HHS/United States
- UC2 HL102924/HL/NHLBI NIH HHS/United States
- RC2 HL103010/HL/NHLBI NIH HHS/United States
- WT_/Wellcome Trust/United Kingdom
- RC2 HL102925/HL/NHLBI NIH HHS/United States
- UC2 HL102925/HL/NHLBI NIH HHS/United States
LinkOut - more resources
Full Text Sources
Other Literature Sources
Miscellaneous