A commensal strain of Staphylococcus epidermidis protects against skin neoplasia - PubMed
- ️Mon Jan 01 2018
A commensal strain of Staphylococcus epidermidis protects against skin neoplasia
Teruaki Nakatsuji et al. Sci Adv. 2018.
Abstract
We report the discovery that strains of Staphylococcus epidermidis produce 6-N-hydroxyaminopurine (6-HAP), a molecule that inhibits DNA polymerase activity. In culture, 6-HAP selectively inhibited proliferation of tumor lines but did not inhibit primary keratinocytes. Resistance to 6-HAP was associated with the expression of mitochondrial amidoxime reducing components, enzymes that were not observed in cells sensitive to this compound. Intravenous injection of 6-HAP in mice suppressed the growth of B16F10 melanoma without evidence of systemic toxicity. Colonization of mice with an S. epidermidis strain producing 6-HAP reduced the incidence of ultraviolet-induced tumors compared to mice colonized by a control strain that did not produce 6-HAP. S. epidermidis strains producing 6-HAP were found in the metagenome from multiple healthy human subjects, suggesting that the microbiome of some individuals may confer protection against skin cancer. These findings show a new role for skin commensal bacteria in host defense.
Figures
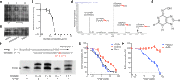
(A and B) Stability of antimicrobial molecules from S. epidermidis against GAS after heat-treatment for the indicated time (A) and incubation with indicated protease (B). The black area represents zone of growth inhibition of GAS. (C) Dose-dependent antimicrobial activity of the purified antimicrobial compound against GAS. Data are means ± SEM of three individual experiments. CFU, colony-forming unit. (D) 15N isotope incorporation into the antibiotic molecule after culturing S. epidermidis MO34 in tryptic soy broth (TSB) containing ammonium-15N chloride (12.5 mM). (E) The determined chemical structure of the active molecule, 6-HAP. (F) Capacity of 6-HAP to block in vitro DNA extension by Klenow fragment polymerase. A template that required adenosine (X = T) or cytidine (X = G) at the initial base for extension was used. (G) 5-Bromo-2′-deoxyuridine (BrdU) incorporation into tumor cell line, L5178, YAC-1 lymphoma, B16F10 melanoma, and Pam212 SCC after a 24-hour incubation in suitable media containing indicated concentrations of 6-HAP. Data are means ± SEM of four individual experiments. (H) BrdU incorporation into nontransformed human keratinocytes (NHEKs) and Pam212 cutaneous squamous cell carcinoma after 24-hour incubation in suitable media containing indicated concentrations of 6-HAP. Data are means ± SEM of four individual experiments.
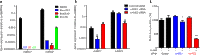
(A) Expression of mARC1 and mARC2 in NHEKs, squamous cell carcinoma (Pam212), melanoma (B16F10), and lymphoma cell lines (L5178). Data are means ± SEM of five individual experiments. UD, undetectable. (B) Expression of mARC1 and mARC2 in NHEKs treated with control siRNA, mARC1 siRNA, and mARC2 siRNA. Data are shown as relative to glyceraldehyde-3-phosphate dehydrogenase (GAPDH) expression and represent means ± SEM of six independent assays. (C) Effect of gene silencing with mARC1 and mARC2 siRNA on sensitivity to 6-HAP in NHEKs. Data are means ± SEM of eight individual experiments (*P < 0.05, ***P < 0.0001 by two-tailed independent t test).
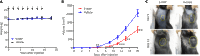
(A) Systemic toxicity of repeated intravascular administration with 6-HAP (20 mg/kg) or with an equal volume of vehicle (2.5% DMSO in 0.9% NaCl) every 48 hours for 2 weeks (arrows) in mice. To observe toxicity of 6-HAP, we determined mouse weight at the indicated time points. Data are means ± SEM of 10 mice. (B and C) Effect of repeated intravascular administrations with 6-HAP on growth of melanoma in mice. Data are means ± SEM from 10 individual mice (*P < 0.05, **P < 0.01, and ***P < 0.001 by two-tailed independent t test versus vehicle control) (B). Representative images of tumor (yellow dashed line) in mouse treated with 6-HAP or vehicle at day 9 and day 13 are shown (C).
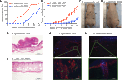
(A to D) Effect of colonization by S. epidermidis MO34 strain producing 6-HAP on tumor incidence (A) and number (B) in SKH-1 hairless mice treated with DMBA, followed by repeated UV-B irradiation. S. epidermidis 1457 was used as a control strain that does not produce 6-HAP. Tumor incidence and tumor number in each mouse were recorded every week. Data are means ± SEM of 19 mice (*P < 0.05, **P < 0.01, and ***P < 0.001 by two-tailed independent t test). Representative images of UV-induced tumor formation in mouse treated with S. epidermidis 1457 (C) or MO34 (D) at week 12 are shown. (E and F) A representative hematoxylin and eosin staining of UV-induced skin tumor or skin obtained from SKH-1 mice colonized by S. epidermidis 1457 (E) or MO34 (F), respectively, treated with UV-B for 12 weeks. (G and H) Immunostaining for S. epidermidis (green) and keratin-14 (red) in the UV-induced tumor or skin of SKH-1 mice treated with S. epidermidis 1457 (G) or MO34 (H), respectively. The sections were counter stained with 4′,6-diamidino-2-phenylindole (blue).
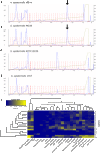
(A to D) Productions of 6-HAP by skin isolate strains, MO34 (A) and MO38 (B), and laboratory strains of S. epidermidis, ATCC12228 (C) and 1457 (D). Production of 6-HAP was evaluated by HPLC. Arrow indicates elution time of 6-HAP. The data are representative of three independent experiments. mAU, milli absorbance units. (E) Heat map showing relative abundance of putative S. epidermidis strains producing 6-HAP in the metagenome of skin microbiome samples from 22 distinct body sites of 18 healthy subjects.
Comment in
-
Kozmin SG, Rogozin IB, Moore EA, Abney M, Schaaper RM, Pavlov YI. Kozmin SG, et al. Sci Adv. 2019 Sep 11;5(9):eaaw3915. doi: 10.1126/sciadv.aaw3915. eCollection 2019 Sep. Sci Adv. 2019. PMID: 31535021 Free PMC article.
Similar articles
-
Kozmin SG, Rogozin IB, Moore EA, Abney M, Schaaper RM, Pavlov YI. Kozmin SG, et al. Sci Adv. 2019 Sep 11;5(9):eaaw3915. doi: 10.1126/sciadv.aaw3915. eCollection 2019 Sep. Sci Adv. 2019. PMID: 31535021 Free PMC article.
-
Hellmann KT, Tuura CE, Fish J, Patel JM, Robinson DA. Hellmann KT, et al. mSphere. 2021 Oct 27;6(5):e0053821. doi: 10.1128/mSphere.00538-21. Epub 2021 Sep 15. mSphere. 2021. PMID: 34523979 Free PMC article.
-
El Farran CA, Sekar A, Balakrishnan A, Shanmugam S, Arumugam P, Gopalswamy J. El Farran CA, et al. Indian J Med Microbiol. 2013 Jan-Mar;31(1):19-23. doi: 10.4103/0255-0857.108712. Indian J Med Microbiol. 2013. PMID: 23508424
-
Staphylococcus epidermidis--the 'accidental' pathogen.
Otto M. Otto M. Nat Rev Microbiol. 2009 Aug;7(8):555-67. doi: 10.1038/nrmicro2182. Nat Rev Microbiol. 2009. PMID: 19609257 Free PMC article. Review.
-
Staphylococcus epidermidis-Skin friend or foe?
Brown MM, Horswill AR. Brown MM, et al. PLoS Pathog. 2020 Nov 12;16(11):e1009026. doi: 10.1371/journal.ppat.1009026. eCollection 2020 Nov. PLoS Pathog. 2020. PMID: 33180890 Free PMC article. Review.
Cited by
-
Licht P, Dominelli N, Kleemann J, Pastore S, Müller ES, Haist M, Hartmann KS, Stege H, Bros M, Meissner M, Grabbe S, Heermann R, Mailänder V. Licht P, et al. NPJ Biofilms Microbiomes. 2024 Aug 29;10(1):74. doi: 10.1038/s41522-024-00542-4. NPJ Biofilms Microbiomes. 2024. PMID: 39198450 Free PMC article.
-
Strains to go: interactions of the skin microbiome beyond its species.
Caldwell R, Zhou W, Oh J. Caldwell R, et al. Curr Opin Microbiol. 2022 Dec;70:102222. doi: 10.1016/j.mib.2022.102222. Epub 2022 Oct 13. Curr Opin Microbiol. 2022. PMID: 36242896 Free PMC article. Review.
-
Causal roles of skin microbiota in skin cancers suggested by genetic study.
Zhu Y, Liu W, Wang M, Wang X, Wang S. Zhu Y, et al. Front Microbiol. 2024 Aug 5;15:1426807. doi: 10.3389/fmicb.2024.1426807. eCollection 2024. Front Microbiol. 2024. PMID: 39161599 Free PMC article.
-
Mud therapy and skin microbiome: a review.
Antonelli M, Donelli D. Antonelli M, et al. Int J Biometeorol. 2018 Nov;62(11):2037-2044. doi: 10.1007/s00484-018-1599-y. Epub 2018 Aug 8. Int J Biometeorol. 2018. PMID: 30091021 Review.
-
Zheng Y, Hunt RL, Villaruz AE, Fisher EL, Liu R, Liu Q, Cheung GYC, Li M, Otto M. Zheng Y, et al. Cell Host Microbe. 2022 Mar 9;30(3):301-313.e9. doi: 10.1016/j.chom.2022.01.004. Epub 2022 Feb 4. Cell Host Microbe. 2022. PMID: 35123653 Free PMC article.
References
-
- Grice E. A., Kong H. H., Conlan S., Deming C. B., Davis J., Young A. C.; NISC Comparative Sequencing Program, Bouffard G. G., Blakesley R. W., Murray P. R., Green E. D., Turner M. L., Segre J. A., Topographical and temporal diversity of the human skin microbiome. Science 324, 1190–1192 (2009). - PMC - PubMed
-
- Byrd A. L., Deming C., Cassidy S. K. B., Harrison O. J., Ng W. I., Conlan S.; NISC Comparative Sequencing Program, Belkaid Y., Segre J. A., Kong H. H., Staphylococcus aureus and Staphylococcus epidermidis strain diversity underlying pediatric atopic dermatitis. Sci. Transl. Med. 9, eaal4651 (2017). - PMC - PubMed
-
- Chng K. R., Tay A. S., Li C., Ng A. H., Wang J., Suri B. K., Matta S. A., McGovern N., Janela B., Wong X. F., Sio Y. Y., Au B. V., Wilm A., De Sessions P. F., Lim T. C., Tang M. B., Ginhoux F., Connolly J. E., Lane E. B., Chew F. T., Common J. E. A., Nagarajan N., Whole metagenome profiling reveals skin microbiome-dependent susceptibility to atopic dermatitis flare. Nat. Microbiol. 1, 16106 (2016). - PubMed
Publication types
MeSH terms
Substances
Grants and funding
LinkOut - more resources
Full Text Sources
Other Literature Sources
Medical