Poligoni Multiflori Radix enhances osteoblast formation and reduces osteoclast differentiation - PubMed
Poligoni Multiflori Radix enhances osteoblast formation and reduces osteoclast differentiation
Sang-Yong Han et al. Int J Mol Med. 2018 Jul.
Abstract
Poligoni Multiflori Radix (PMR) is a traditional Korean medicinal herb that is known to have various pharmacological effects, including antihyperlipidemic, anticancer, and anti‑inflammatory effects. However, the effects of PMR on bone metabolism have not been elucidated to date. The present study aimed to investigate the in vitro and in vivo effect of PMR water extract on the regulation of osteoblast and osteoclast activity. Effects of PMR water extract on receptor activator of nuclear factor‑kB ligand (RANKL)‑induced osteoclast differentiation and survival of mouse bone marrow macrophages (BMMs) obtained from femurs were investigated by tartrate‑acid resistant acid phosphatase (TRAP)‑positive cells and XTT assay. Expression of osteoclast‑related genes was assayed by western blot analysis and reverse transcription‑quantitative polymerase chain reaction. Additionally, the effects of PMR water extract on osteoblastic proliferation and differentiation were investigated by alkaline phosphatase (ALP) activity assay, alizarin red staining, and levels of mRNA encoding known osteoblast markers. Furthermore, the effects of PMR water extract on lipopolysaccharide (LPS)‑induced bone loss were examined in a mouse model. PMR inhibited RANKL‑induced osteoclast differentiation of BMMs in a dose‑dependent manner without significant cytotoxicity, and suppressed expression of the main osteoclast differentiation markers Fos proto‑oncogene and nuclear factor of activated T‑cell. In addition, PMR decreased the mRNA expression levels of NFATc1 target genes, including TRAP, osteoclast‑associated receptor, ATPase H+ transporting, lysosomal 38 kDa V0 subunit d2, and Cathepsin K. These inhibitory effects were mediated by the p38 and extracellular signal‑regulated kinase/nuclear factor‑κB pathway. Simultaneously, PMR enhanced the differentiation of primary osteoblasts, and increased the mRNA expression of runt‑related transcription factor 2, ALP, osterix, and osteocalcin. Notably, PMR improved LPS‑induced trabecular bone loss in mice. Collectively, the present findings demonstrated that PMR may regulate bone remodeling by reducing osteoclast differentiation and stimulating osteoblast formation. These results suggest that PMR may be used for the treatment of bone diseases, such as osteoporosis and rheumatoid arthritis.
Figures
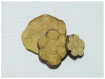
Photograph of PMR used in the present study. In Korea, PMR is frequently confused with other herb with similar Korean name, Cynanchum wilfordii Radix. PMR, Polygoni Multiflori Radix.
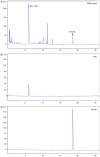
Ultra-performance liquid chromatography chromatograms of PMR and standard compounds. (A) PMR. (B) THS. (C) Emodin. PMR, Polygoni Multiflori Radix; THS, 2,3,5,4′-tetrahydroxystilbene 2-O-β-D-glucoside.
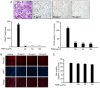
Effects of PMR on RANKL-induced osteoclast differentiation in BMMs. BMMs were cultured with vehicle or PMR (25, 50 and 100 µg/ml) in the presence of M-CSF (30 ng/ml) and RANKL (100 ng/ml) for 4 days. (A) Cultured cells were fixed and stained for TRAP. TRAP activity was determined by measuring optical density values at 450 nm. TRAP-positive cells containing three or more nuclei were counted as MNCs. (B) Cellular morphology of F-actin rings were observed by immunofluorescence. F-actin rings were stained by Texas-red phalloidin (red signal) and nuclei were stained by DAPI (blues signal). (C) Cell viability of BMMs in the presence of PMR and M-CSF (30 ng/ml) was determined using the XTT assay. ***P<0.001 vs. control group. PMR, Polygoni Multiflori Radix; RANKL, receptor activator of nuclear factor-kB ligand; BMMs, bone marrow macrophages; M-CSF, macrophage colony stimulating factor; TRAP, tartrate-acid resistant acid phosphatase; MNCs, multinuclear osteoclast cells.
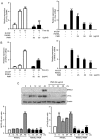
Effects of PMR on the RANKL-induced expression of c-Fos and NFATc1 in BMMs. BMMs were treated with PMR and then stimulated with M-CSF (30 ng/ml) and RANKL (100 ng/ml) at the indicated time periods and concentrations. (A) The mRNA expression levels of c-Fos and (B) NFATc1 were analyzed by reverse transcription-quantitative polymerase chain reaction. (C) Western blot analysis was performed to confirm the results at the protein level. β-actin was used as an internal control. **P<0.01 and ***P<0.001 vs. control group; ##P<0.01 and ###P<0.001 vs. RANKL-treated group at the corresponding time and concentration. PMR, Polygoni Multiflori Radix; RANKL, receptor activator of nuclear factor-kB ligand; c-Fos, Fos proto-oncogene; NFATc1, nuclear factor of activated T-cell; BMMs, bone marrow macrophages; M-CSF, macrophage colony stimulating factor.
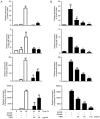
(A and B) PMR inhibits the expression of osteoclast-specific genes. BMMs were stimulated with M-CSF (30 ng/ml) and RANKL (100 ng/ml) in the presence or absence of PMR for the indicated time periods and indicated concentrations. The mRNA expression levels of TRAP, OSCAR, ATP6vod2 and Cathepsin K were analyzed by reverse transcription-quantitative polymerase chain reaction. **P<0.01 and ***P<0.001 vs. control group; #P<0.05, ##P<0.01 and ###P<0.001 vs. RANKL-treated group at the corresponding time and concentration. PMR, Polygoni Multiflori Radix; BMMs, bone marrow macrophages; M-CSF, macrophage colony stimulating factor; RANKL, receptor activator of nuclear factor-kB ligand; TRAP, tartrate-acid resistant acid phosphatase; OSCAR, osteoclast-associated receptor; ATP6vod2, ATPase H+ transporting lysosomal 38 kDa V0 subunit d2.
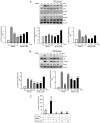
PMR inhibits the RANKL-mediated early signaling in osteoclast differentiation. (A and B) BMMs were incubated without serum for 2 h and pretreated with PMR (50 µg/ml) for 1 h. RANKL (100 ng/ml) was added for the indicated time. The cell lysates were analyzed by western blotting with the indicated antibodies. β-actin was used as an internal control. (C) 293T cells were co-transfected with TRAF6 and NF-κB-luciferase reporter plasmid. Following transfection, the cells were treated with PMR (25, 50 and 100 µg/ml) or vehicle for 24 h. The cells were lysed and used for the luciferase activity. *P<0.05, **P<0.01 and ***P<0.001 vs. control group; ##P<0.01 and ###P<0.001 vs. RANKL-treated group at the corresponding time and concentration. PMR, Polygoni Multiflori Radix; RANKL, receptor activator of nuclear factor-kB ligand; BMMs, bone marrow macrophages; TRAF6, TNF receptor-associated factor 6; NF-κB, nuclear factor-κB.
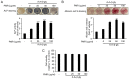
Effects of PMR on the AA/β-GP-induced osteoblast differentiation in primary mouse osteoblasts. Primary mouse osteoblasts were seeded in 48-well plates at a density 1.5×104 cells/well and cultured in the absence or presence of β-GP (10 mM) and AA (50 µg/ml). (A) Primary osteoblasts were treated with PMR for 7 days. ALP positive cells were detected by ALP staining (upper panel). ALP activity is expressed as amount of p-nitrophenol released (lower panel). (B) Bone nodule mineralization of primary osteoblasts determined by alizarin red S staining after 21 days (upper panel). The intensity of staining was quantified with cetylpyridium chloride solution (lower panel). (C) Cell viability was determined by the XTT assay. *P<0.05, **P<0.01 and ***P<0.001 vs. control. PMR, Polygoni Multiflori Radix; AA, ascorbic acid; β-GP, β-glycerophosphate; ALP, alkaline phosphatase.
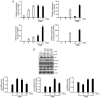
Effect of PMR on osteoblast-specific markers and on the p38 and Smad pathways. (A) Primary mouse osteoblasts were seeded in 6-well plates at a density 2×105 cells/well and cultured in the absence or presence of β-GP (10 mM) and AA (50 µg/ml) for the indicated days. Levels of mRNA expression of Runx2, ALP, osterix and osteocalcin were analyzed by reverse transcription-quantitative polymerase chain reaction. (B) Primary mouse osteoblasts were serum-starved for 2 h, and treated with PMR (50 µg/ml) for the indicated time. The cell lysates were analyzed by western blotting with the indicated antibodies. β-actin was used as an internal control. *P<0.05, **P<0.01 and ***P<0.001 vs. control group; #P<0.05, ##P<0.01 and ###P<0.001 vs. AA/β-GP-induced group at the corresponding time. PMR, Polygoni Multiflori Radix; AA, ascorbic acid; β-GP, β-glycerophosphate; Runx2, runt-related transcription factor 2; ALP, alkaline phosphatase; p-, phosphorylated; ERK, extracellular signal-regulated kinase.
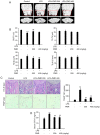
Effect of PMR on LPS-induced bone loss in vivo. (A) Representative three-dimensional reconstruction images of femurs from high-resolution µCT analysis. (B) The BV/TV, Tb.Sp, Tb.Th, and Tb.N of the femurs were determined using the µCT data, analyzed by Skyscan 1172 software. (C) Dissected femora were fixed, decalcified, embedded in paraffin and sectioned. Sections were stained with H&E (upper images) and with TRAP (lower images). The number of osteoclasts per field was counted using the histomorphometric results. (D) Serum levels of CTX-1 were determined using a mouse ELISA kit. *P<0.05, **P<0.01, ***P<0.001 vs. control group; #P<0.05 and ##P<0.01 vs. LPS-treated group. PMR, Polygoni Multiflori Radix; LPS, lipopolysaccharide; µCT, micro-computed tomography; BV/TV, trabecular bone volume/total tissue volume; Tb.Sp, trabecular separation; Tb.Th, trabecular thickness; Tb.N, trabecular number; H&E, hematoxylin and eosin; TRAP, tartrate-acid resistant acid phosphatase. CTX-1, C-teminal telopeptide of type I collagen.
Similar articles
-
Tran PT, Park DH, Kim O, Kwon SH, Min BS, Lee JH. Tran PT, et al. Int J Mol Med. 2018 Jul;42(1):569-578. doi: 10.3892/ijmm.2018.3627. Epub 2018 Apr 17. Int J Mol Med. 2018. PMID: 29693149
-
Han SY, Kim YK. Han SY, et al. Am J Chin Med. 2019;47(2):439-455. doi: 10.1142/S0192415X19500228. Epub 2019 Mar 4. Am J Chin Med. 2019. PMID: 30827151
-
The Role of Rosavin in the Pathophysiology of Bone Metabolism.
Wojdasiewicz P, Turczyn P, Lach-Gruba A, Poniatowski ŁA, Purrahman D, Mahmoudian-Sani MR, Szukiewicz D. Wojdasiewicz P, et al. Int J Mol Sci. 2024 Feb 9;25(4):2117. doi: 10.3390/ijms25042117. Int J Mol Sci. 2024. PMID: 38396794 Free PMC article. Review.
-
Osteoblast-osteoclast interactions.
Chen X, Wang Z, Duan N, Zhu G, Schwarz EM, Xie C. Chen X, et al. Connect Tissue Res. 2018 Mar;59(2):99-107. doi: 10.1080/03008207.2017.1290085. Epub 2017 Mar 21. Connect Tissue Res. 2018. PMID: 28324674 Free PMC article. Review.
Cited by
-
Lee J, Ahn SH, Chen Z, Kang S, Choi DK, Moon C, Min SH, Park BJ, Lee TH. Lee J, et al. Molecules. 2020 Oct 21;25(20):4855. doi: 10.3390/molecules25204855. Molecules. 2020. PMID: 33096734 Free PMC article.
-
Guo DY, Chen ZH, Fu YF, Li YY, Chen MN, Wu JJ, Yuan ZD, Ye JX, Li X, Yuan FL. Guo DY, et al. Heliyon. 2023 Jun 29;9(7):e17841. doi: 10.1016/j.heliyon.2023.e17841. eCollection 2023 Jul. Heliyon. 2023. PMID: 37539209 Free PMC article.
-
Lipopolysaccharide-Induced Bone Loss in Rodent Models: A Systematic Review and Meta-Analysis.
Bott KN, Feldman E, de Souza RJ, Comelli EM, Klentrou P, Peters SJ, Ward WE. Bott KN, et al. J Bone Miner Res. 2023 Jan;38(1):198-213. doi: 10.1002/jbmr.4740. Epub 2022 Dec 5. J Bone Miner Res. 2023. PMID: 36401814 Free PMC article.
-
Ren GW, Wen SB, Han J, Xu ZW, Qi W, Shang YZ, Wu YK. Ren GW, et al. Biomed Res Int. 2022 Jul 23;2022:8080679. doi: 10.1155/2022/8080679. eCollection 2022. Biomed Res Int. 2022. PMID: 35915795 Free PMC article.
References
MeSH terms
Substances
LinkOut - more resources
Full Text Sources
Other Literature Sources
Miscellaneous