Stress and stem cells: adult Muse cells tolerate extensive genotoxic stimuli better than mesenchymal stromal cells - PubMed
- ️Mon Jan 01 2018
Stress and stem cells: adult Muse cells tolerate extensive genotoxic stimuli better than mesenchymal stromal cells
Nicola Alessio et al. Oncotarget. 2018.
Abstract
Mesenchymal stromal cells (MSCs) are not a homogenous population but comprehend several cell types, such as stem cells, progenitor cells, fibroblasts, and other types of cells. Among these is a population of pluripotent stem cells, which represent around 1-3% of MSCs. These cells, named multilineage-differentiating stress enduring (Muse) cells, are stress-tolerant cells. Stem cells may undergo several rounds of intrinsic and extrinsic stresses due to their long life and must have a robust and effective DNA damage checkpoint and DNA repair mechanism, which, following a genotoxic episode, promote the complete recovery of cells rather than triggering senescence and/or apoptosis. We evaluated how Muse cells can cope with DNA damaging stress in comparison with MSCs. We found that Muse cells were resistant to chemical and physical genotoxic stresses better than non-Muse cells. Indeed, the level of senescence and apoptosis was lower in Muse cells. Our results proved that the DNA damage repair system (DDR) was properly activated following injury in Muse cells. While in non-Muse cells some anomalies may have occurred because, in some cases, the activation of the DDR persisted by 48 hr post damage, in others no activation took place. In Muse cells, the non-homologous end joining (NHEJ) enzymatic activity increases compared to other cells, while single-strand repair activity (NER, BER) does not. In conclusion, the high ability of Muse cells to cope with genotoxic stress is related to their quick and efficient sensing of DNA damage and activation of DNA repair systems.
Keywords: apoptosis; mesenchymal stem cells; senescence.
Conflict of interest statement
CONFLICTS OF INTEREST None.
Figures
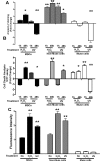
(A, B) Apoptosis and global cell death analysis, performed with Annexin V and formazan assays, respectively. Data are expressed as fold changes with standard error (± SD, n = 3, *p < 0.05, **p < 0.05). For each cell type, in every experimental condition, the apoptosis or global cell death level in samples not treated with genotoxic agents (H2O2 or UV) is set as the baseline (zero value). Increase or decrease of apoptosis or cell death in treated samples is shown as columns above or below the control baselines, respectively. (C) Acid beta-galactosidase senescence assay. The graph shows senescent level in every experimental condition (± SD, n = 3, *p < 0.05). Data expressed as arbitrary units (fluorescence intensity).
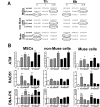
MSCs, non-Muse cells, and-Muse cells were treated with H202 or UV. (A) - The table shows the cell cycle analysis 1, 6, and 48 hr following treatments. Data are expressed in percentage (± SD, n = 3). In treated samples, the values in the ovals are statistically different (p < 0.05) from corresponding controls. (B) - The graphs show results from the immunostaining analysis of proteins involved in DNA repair signaling 1, 6, and 48 hr following treatments. The mean percentages of ATM, RAD51, and DNA-PK positive cells are reported (± SD, n = 3, *p < 0.05, **p < 0.05).
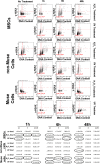
MSCs, non-Muse cells, and-Muse cells were treated with H202 or UV. At 1, 6, and 48 hr following treatments, a flow cytometry analysis of γ-H2AX protein, combined with propidium iodide DNA staining, was performed to detect foci of damaged DNA in the different phases of the cycle. For every experimental condition, the percentages of γ-H2AX-positive cells are indicated in the table. Data are expressed with standard deviation (n = 3). In treated samples, the values in the ovals are statistically different (p < 0.05) from corresponding controls.
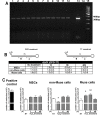
MSCs, non-Muse cells, and-Muse cells were treated with UV (A, B) or H202 (C). 1, 6, and 48 hr following treatments, several enzymatic assays were carried out for evaluation of DNA repair abilities. Panel A: A 347 bp fragment of human GAPDH gene was amplified by PCR and analyzed by agarose gel electrophoresis following incubation with cell lysates. DNA amplicons contained uridine deoxynucleotides and could be degraded by uracil DNA glycosidase present in cell extracts. This enzymatic activity was indirectly determined by calculating the ratio between pixel intensities of intact DNA bands coming from cell lysate- treated and untreated amplicons. In the panel is reported an exemplificative agarose gel. GAPDH amplicons were incubated with untreated MSCs (lane 1) or with MSC cell extracts obtained 1 hr (lane 4), 6 hr (lane 7), 48 hr (lane 10) following UV irradiation. Same experimental scheme was applied for non-Muse cells (lanes 2, 5, 8, 11) and Muse cells (lanes 3, 6, 9, 12). Lane 13: GAPDH amplicon not incubated with cell extracts (BER negative control); lane 14: GAPDH amplicon treated with uracil DNA glycosidase (BER positive control); lane 15: DNA molecular weight marker. (B) Two different DNA constructs with a hairpin duplex structure (CPD and TT construct, respectively) were used as a template for qPCR amplification (see inset). CPD and TT constructs differ only in a region that has either a normal TT couple (TT construct) or a cyclobutane pyrimidine dimer (CPD construct). Two primers, named A and C, encompassed a region containing cyclobutane pyrimidine dimers in the CPD construct and a normal TT couple in the TT construct. The presence of these dimers in the CPD construct impaired activity of DNA polymerase during PCR unless these lesions were repaired by the NER system. Two other primers, named A and B, encompassed a DNA region that did not contain dimers in both constructs and served as controls of PCR efficiency. The constructs were incubated with cell lysates from Muse cells, non-Muse cells, and MSCs. In these conditions, the NER activity of cell extracts might repair a cyclobutane dimer and increase PCR yield in samples containing the CPD construct as templates. The incubation of the TT construct with cell lysate did not further increase PCR products. Data analysis were performed with the following equation: DCt = Ct (A/C primers) – Ct (A/B primers). The DCt was determined for TT construct, used as reference, and for CPD construct. We calculated the NER activity in each experimental condition with DDCt value (CPD – TT construct). The table reports the NER activity before (No treatment) and after (1, 6, 48 hr) UV treatment (± SD, n = 3). (C) The p100048 plasmid was digested with an Age I enzyme and used as the template for a qPCR reaction that amplifies a 135 bp fragment encompassing the region containing the Age I restriction site. Digested plasmids were incubated with cell lysates from Muse cells, non-Muse cells, and MSCs. In these conditions, the NHEJ activity of cell extracts might end-join plasmids and allow PCR amplification. Different amounts of undigested plasmids were amplified to create a standard curve with Ct (threshold cycle). This allowed us to obtain a relative measure of the amount of amplicon in the different experimental conditions. The higher the quantity of obtained amplicon from digested plasmid templates, the better the NHEJ activity. The graphs named MSCs, non-Muse cells and Muse cells report the number of amplified molecules before (NT) and after (1, 6, 48 hr) H2O2 treatment (± SD, n = 3, *p < 0.05, **p < 0.01). Positive control shows the number of amplified molecules obtained from undigested plasmid.
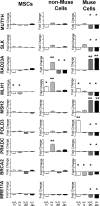
The histograms show the quantitative RT-PCR analysis of a group of genes involved in BER, NER, MMR, NHEJ, and HR. The mRNA levels were normalized to GAPDH mRNA expression, which was selected as an internal control. Histograms show expression levels in the different conditions. Data are expressed as fold changes with standard error (± SD, n = 3, *p < 0.05). For each gene in every experimental condition, the expression level in not-differentiated samples is set as the baseline (one value). Up- or down-regulation of genes in differentiated samples is shown as columns above or below the control baselines, respectively.
Similar articles
-
Stem Cells and DNA Repair Capacity: Muse Stem Cells Are Among the Best Performers.
Squillaro T, Alessio N, Di Bernardo G, Özcan S, Peluso G, Galderisi U. Squillaro T, et al. Adv Exp Med Biol. 2018;1103:103-113. doi: 10.1007/978-4-431-56847-6_5. Adv Exp Med Biol. 2018. PMID: 30484225 Review.
-
Alessio N, Özcan S, Tatsumi K, Murat A, Peluso G, Dezawa M, Galderisi U. Alessio N, et al. Cell Cycle. 2017 Jan 2;16(1):33-44. doi: 10.1080/15384101.2016.1211215. Epub 2016 Jul 27. Cell Cycle. 2017. PMID: 27463232 Free PMC article.
-
Acar MB, Aprile D, Ayaz-Guner S, Guner H, Tez C, Di Bernardo G, Peluso G, Ozcan S, Galderisi U. Acar MB, et al. Int J Mol Sci. 2021 Feb 19;22(4):2064. doi: 10.3390/ijms22042064. Int J Mol Sci. 2021. PMID: 33669748 Free PMC article.
-
The Muse Cell Discovery, Thanks to Wine and Science.
Dezawa M. Dezawa M. Adv Exp Med Biol. 2018;1103:1-11. doi: 10.1007/978-4-431-56847-6_1. Adv Exp Med Biol. 2018. PMID: 30484221 Review.
-
Dezawa M. Dezawa M. Cell Transplant. 2016;25(5):849-61. doi: 10.3727/096368916X690881. Epub 2016 Feb 15. Cell Transplant. 2016. PMID: 26884346 Review.
Cited by
-
Xu P, Chang J, Ma G, Liao F, Xu T, Wu Y, Yin Z. Xu P, et al. BMC Musculoskelet Disord. 2022 Nov 26;23(1):1020. doi: 10.1186/s12891-022-05928-z. BMC Musculoskelet Disord. 2022. PMID: 36435763 Free PMC article.
-
Yamashita T, Kushida Y, Abe K, Dezawa M. Yamashita T, et al. Cells. 2021 Apr 20;10(4):961. doi: 10.3390/cells10040961. Cells. 2021. PMID: 33924240 Free PMC article. Review.
-
MicroRNA-18a prevents senescence of mesenchymal stem cells by targeting CTDSPL.
Sun B, Meng XH, Li YM, Lin H, Xiao ZD. Sun B, et al. Aging (Albany NY). 2024 Mar 7;16(5):4904-4919. doi: 10.18632/aging.205642. Epub 2024 Mar 7. Aging (Albany NY). 2024. PMID: 38460957 Free PMC article.
-
Shirakawa J, Ntege EH, Takemura M, Miyamoto S, Kawano T, Sampei C, Kawabata H, Nakamura H, Sunami H, Hayata T, Shimizu Y. Shirakawa J, et al. Regen Ther. 2024 May 25;26:71-79. doi: 10.1016/j.reth.2024.05.004. eCollection 2024 Jun. Regen Ther. 2024. PMID: 38828011 Free PMC article.
-
Human adult pluripotency: Facts and questions.
Labusca L, Mashayekhi K. Labusca L, et al. World J Stem Cells. 2019 Jan 26;11(1):1-12. doi: 10.4252/wjsc.v11.i1.1. World J Stem Cells. 2019. PMID: 30705711 Free PMC article.
References
-
- Galderisi U, Giordano A. The gap between the physiological and therapeutic roles of mesenchymal stem cells. Med Res Rev. 2014;34:1100–26. https://doi.org/10.1002/med.21322. - DOI - PubMed
-
- Wakao S, Kuroda Y, Ogura F, Shigemoto T, Dezawa M. Regenerative Effects of Mesenchymal Stem Cells: Contribution of Muse Cells, a Novel Pluripotent Stem Cell Type that Resides in Mesenchymal Cells. Cells. 2012;1:1045–60. https://doi.org/10.3390/cells1041045. - DOI - PMC - PubMed
-
- Wakao S, Kitada M, Kuroda Y, Shigemoto T, Matsuse D, Akashi H, Tanimura Y, Tsuchiyama K, Kikuchi T, Goda M, Nakahata T, Fujiyoshi Y, Dezawa M. Multilineage-differentiating stress-enduring (Muse) cells are a primary source of induced pluripotent stem cells in human fibroblasts. Proc Natl Acad Sci U S A. 2011;108:9875–80. https://doi.org/10.1073/pnas.1100816108. - DOI - PMC - PubMed
-
- Dezawa M. Muse cells provide the pluripotency of mesenchymal stem cells: direct contribution of Muse cells to tissue regeneration. Cell Transplant. 2016;25:849–61. https://doi.org/10.3727/096368916X690881. - DOI - PubMed
-
- Tsuchiyama K, Wakao S, Kuroda Y, Ogura F, Nojima M, Sawaya N, Yamasaki K, Aiba S, Dezawa M. Functional melanocytes are readily reprogrammable from multilineage-differentiating stress-enduring (muse) cells, distinct stem cells in human fibroblasts. J Invest Dermatol. 2013;133:2425–35. https://doi.org/10.1038/jid.2013.172. - DOI - PubMed
LinkOut - more resources
Full Text Sources
Other Literature Sources