Cholinergic excitation complements glutamate in coding visual information in retinal ganglion cells - PubMed
. 2018 Aug;596(16):3709-3724.
doi: 10.1113/JP275073. Epub 2018 Jun 21.
Affiliations
- PMID: 29758086
- PMCID: PMC6092279
- DOI: 10.1113/JP275073
Cholinergic excitation complements glutamate in coding visual information in retinal ganglion cells
Santhosh Sethuramanujam et al. J Physiol. 2018 Aug.
Abstract
Starburst amacrine cells release GABA and ACh. This study explores the coordinated function of starburst-mediated cholinergic excitation and GABAergic inhibition to bistratified retinal ganglion cells, predominantly direction-selective ganglion cells (DSGCs). In rat retina, under our recording conditions, starbursts were found to provide the major excitatory drive to a sub-population of ganglion cells whose dendrites co-stratify with starburst dendrites (putative DSGCs). In mouse retina, recordings from genetically identified DSGCs at physiological temperatures reveal that ACh inputs dominate the response to small spot-high contrast light stimuli, with preferential addition of bipolar cell input shifting the balance towards glutamate for larger spot stimuli In addition, starbursts also appear to gate glutamatergic excitation to DSGCs by postsynaptic and possibly presynaptic inhibitory processes ABSTRACT: Starburst amacrine cells release both GABA and ACh, allowing them to simultaneously mediate inhibition and excitation. However, the precise pre- and postsynaptic targets for ACh and GABA remain under intense investigation. Most previous studies have focused on starburst-mediated postsynaptic GABAergic inhibition and its role in the formation of directional selectivity in ganglion cells. However, the significance of postsynaptic cholinergic excitation is only beginning to be appreciated. Here, we found that light-evoked responses measured in bi-stratified rat ganglion cells with dendrites that co-fasciculate with ON and OFF starburst dendrites (putative direction-selective ganglion cells, DSGCs) were abolished by the application of nicotinic receptor antagonists, suggesting ACh could act as the primary source of excitation. Recording from genetically labelled DSGCs in mouse retina at physiological temperatures revealed that cholinergic synaptic inputs dominated the excitation for high contrast stimuli only when the size of the stimulus was small. Canonical glutamatergic inputs mediated by bipolar cells were prominent when GABA/glycine receptors were blocked or when larger spot stimuli were utilized. In mouse DSGCs, bipolar cell excitation could also be unmasked through the activation of mGluR2,3 receptors, which we show suppresses starburst output, suggesting that GABA from starbursts serves to inhibit bipolar cell signals in DSGCs. Taken together, these results suggest that starbursts amplify excitatory signals traversing the retina, endowing DSGCs with the ability to encode fine spatial information without compromising their ability to encode direction.
Keywords: acetylcholine; feedback inhibition; starburst amacrine cells.
© 2018 The Authors. The Journal of Physiology © 2018 The Physiological Society.
Figures
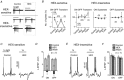
A, the light responses of two ON‐OFF and an ON sustained retinal ganglion cell under control conditions, then in the presence of 100 μM hexamethonium (HEX). The top ON‐OFF cell was designated HEX‐sensitive, as its spiking was blocked completely by HEX (see text for details). The spiking in the lower two cells was unaffected, and designated HEX‐insensitive. The 1s light stimulus, a 200 μm diameter green spot (2.4 × 104 R*/rod/s), is represented by the solid bar above each trace. B, summary of relative number of spikes in HEX‐sensitive and HEX‐insensitive neurons in control, then in the presence of HEX, after recovery, and in the presence of
d‐tubocurarine (Tubo) (* P < 0.005). HEX‐sensitive neurons were all ON‐OFF cells (n = 20), while HEX‐insensitive neurons were either ON‐OFF transient (n = 20), ON sustained (n = 9) or OFF sustained (n = 9). C, the L‐EPSP of a HEX‐sensitive neuron recorded in whole cell configuration in control and HEX. D, the average L‐EPSP (spikes not included) of HEX‐sensitive cells (n = 7) in control, HEX and wash (* P < 0.005). E, the L‐EPSPs of a HEX‐insensitive neuron in control and HEX. F, the average L‐EPSP in HEX‐insensitive neurons in control, HEX and wash (n = 7).
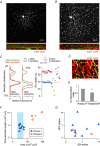
A and B, dendritic stratification of Alexa 488‐stained cells that co‐stratify with starburst dendrites labelled with ChAT antibodies (bottom panel). Scale bar = 50 μm. C, relative fluorescence of starburst dendrites along the depth of the IPL compared to the dendrites of a HEX‐sensitive cell (left) and a HEX‐insensitive neuron (middle). The right panel shows the stratification index (see methods) of 13 HEX‐sensitive cells and 11 HEX‐insensitive cells. The dashed line indicates the average ON (38 ± 1%) and OFF (68 ± 1%) ChAT bands (n = 24). The ON and OFF arbors were not clear for the two ON‐OFF HEX‐insensitive cells and hence are represented by a single value. D, the co‐fasciculation of a HEX‐sensitive neuron's dendrites with the starburst dendrites (scale bar = 50 μm). E, summary of the average co‐fasciculation index for all HEX‐sensitive cells compared to when the index was calculated after rotating the HEX‐sensitive cell's dendrites by 180° (see methods; 0° = 0.77 ± 0.02; 180° = 0.54 ± 0.03; * P < 0.001). F, a plot of the total dendritic length compared to dendritic area in HEX‐sensitive cells. Cluster analysis indicated two groups of HEX‐sensitive cells (n = 12). The (+) symbols indicate the centres of the two groups. The light shaded region shows the dendritic area of DSGC‐like cells observed by Sun et al. (2002). Hence Group 1 cells are likely to be DSGCs. We were unable to reconstruct the complete dendritic tree for one HEX‐sensitive cell, which was excluded from this analysis. G, comparison of the total number of OFF spikes vs. ON spikes evoked by light shown in Fig. 1. The two groups could not be differentiated based on this property.
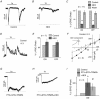
A and B, light evoked excitatory postsynaptic currents (L‐EPSC) of a rat HEX‐sensitive neuron in control and in 100 μM HEX. The light stimulus was a 200 μm diameter green spot with an intensity of 2.4 × 104 R*/rod/s. C, mean relative peak of ON and OFF L‐EPSCs in HEX relative to control and wash (n = 11; * P < 0.001). D, the light‐evoked inhibitory postsynaptic currents (L‐IPSCs) of a HEX‐sensitive neuron in control and in HEX. E, mean relative peak of ON and OFF L‐IPSCs in HEX relative to control (n = 7; ON: P = 0.37; OFF: P = 0.26). F, mean peak amplitude of postsynaptic currents in HEX‐sensitive neurons held at seven different voltages (−90 mV to +30 mV), in control and HEX (n = 3). The inset shows the ACh component estimated as the difference of currents in control and HEX. G and H, the L‐EPSCs of the cell shown in A in PTX + STR + TPMPA, then plus HEX. PTX + STR + TPMPA dramatically increased the L‐EPSCs; note the change in scale. I, peak ON L‐EPSCs in PTX + STR + TPMPA and then plus HEX relative to control (n = 6; * P < 0.005, # P < 0.05).
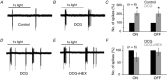
A and B, the light evoked spike activity of a rat HEX‐sensitive neuron in control and 10 μM DCG‐IV (200 μm diameter green spot; 2.4 × 104 R*/rod/s). C, the mean spike count of six cells in DCG‐IV relative to control (* P < 0.05). D and E, the light evoked spike activity of a HEX‐sensitive neuron in DCG‐IV and then DCG‐IV plus HEX. Under control conditions, HEX completely blocked the spiking in these cells (data not shown). F, the mean spike count of five cells in DCG + HEX relative to DCG (ON: P = 0.21, OFF: P = 0.72).
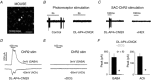
A, YFP/GFP expression in the ganglion cell layer of Hb9 × Chat‐Cre:ChR2 mouse retina. Chat‐positive neurons (starbursts) were YFP labelled and Hb9 neurons (DSGCs) expressed GFP (arrowhead). Scale bar = 50 μm. B, the light‐evoked photoreceptor‐mediated spike responses of an Hb9 cell in Chat‐Cre:ChR2 mouse retina, in control (left) and in the presence of
dl‐AP4 + CNQX (right). A 200 μm diameter, white spot of intensity 20 R*/rod/s was used to elicit photoreceptor‐mediated responses. C, the response of the same cell when ChR2 was stimulated using a blue LED (108 R*/rod/s; left; n = 8). The ChR2‐stimulated spiking could be blocked by HEX (right; n = 4). D, the currents generated in a voltage clamped Hb9 neuron held at −60 mV (EPSC; ACh) and 0 mV (IPSC; GABA) in response to ChR2 stimulation in
dl‐AP4 + CNQX. E, in the same cell addition of DCG blocked both the ACh and the GABA currents. F, summary of the mean peak GABA currents (left) and ACh currents (right) in the presence and absence of DCG (n = 6; P < 0.005*).
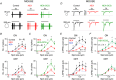
A, the ON and OFF spiking of a GFP+ cell in the Hb9 mouse retina in response to 50 and 200 μm diameter white spots (intensity = 20 R*/rod/s) under control conditions, then in the presence of HEX, and then in HEX + DCG. B, summary of the number of spikes in the ON (top) and OFF (bottom) responses with different spot sizes in control and HEX. Spike activity was significantly reduced in HEX compared to control (n = 6, * P < 0.05). The ACh component was estimated as the difference of spiking in control and HEX (dashed line). C, summary of the average number of spikes in the ON (top) and OFF (bottom) responses with different spot sizes in HEX and HEX + DCG. Spike activity was significantly increased in DCG (n = 6, * P < 0.01). D, the L‐EPSCs of an Hb9 neuron in response to 50 and 200 μm diameter spots of light in control, HEX and HEX + DCG. E and F, summary of the mean peak ON (top) and OFF (bottom) L‐EPSCs to the protocol shown in D (n = 7, * P < 0.05).
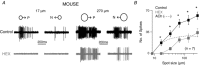
A, the spike response of an Hb9 neuron to 17 and 270 μm diameter moving white spots in the preferred and null direction (1 mm/s; intensity = 20 R*/rod/s), under control conditions and in HEX. B, the average number of spikes of the preferred direction responses in the conditions shown in A (n = 7; * P < 0.005). The ACh component was estimated as the difference of spiking in control and HEX (dashed line).
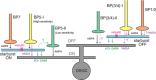
The ON starbursts and ON dendrites of DSGCs receive depolarizing glutamate excitation (i.e. AMPA receptor activity) from distinct bipolar cells (Poleg‐Polsky & Diamond, 2016; Sethuramanujam et al. 2017). BP5‐I, a bipolar cell with high‐contrast sensitivity, probably provides AMPA receptor‐mediated excitation to ON starbursts while providing NMDA receptor‐mediated excitation to ON dendrites of DSGCs (Sethuramanujam et al. 2017). BP5‐II, a low‐sensitivity bipolar cell, provides AMPA receptor‐mediated excitation exclusively to DSGC ON dendrites. Furthermore, BP7 provides exclusive AMPA receptor‐mediated inputs to starbursts (Helmstaedter et al. 2013). In the OFF pathway, BP1/2 and BP(3/4)‐I provide excitation to starbursts via AMPA and NMDA receptors (Fransen & Borghuis, 2017). BP(3/4)‐I also provides excitation to DSGCs (Helmstaedter et al. 2013), probably via NMDA receptors to ensure robust direction computation (Sethuramanujam et al. 2017). BP(3/4)‐II provides exclusive excitation to DSGCs via AMPA receptors and possibly via NMDA receptors. The OFF NMDA receptor pathways require further confirmation and hence are indicated by a dashed line. Both starbursts provide ACh excitation and GABA inhibition to DSGCs. Starbursts express mGluR2 receptors, which upon activation reduce ACh/GABA release. Furthermore, the ON starbursts seem to provide GABA inhibition to BP5‐II, a circuit feature absent in the OFF pathway.
Similar articles
-
Park SJ, Kim IJ, Looger LL, Demb JB, Borghuis BG. Park SJ, et al. J Neurosci. 2014 Mar 12;34(11):3976-81. doi: 10.1523/JNEUROSCI.5017-13.2014. J Neurosci. 2014. PMID: 24623775 Free PMC article.
-
Pei Z, Chen Q, Koren D, Giammarinaro B, Acaron Ledesma H, Wei W. Pei Z, et al. J Neurosci. 2015 Sep 23;35(38):13219-32. doi: 10.1523/JNEUROSCI.0933-15.2015. J Neurosci. 2015. PMID: 26400950 Free PMC article.
-
A Central Role for Mixed Acetylcholine/GABA Transmission in Direction Coding in the Retina.
Sethuramanujam S, McLaughlin AJ, deRosenroll G, Hoggarth A, Schwab DJ, Awatramani GB. Sethuramanujam S, et al. Neuron. 2016 Jun 15;90(6):1243-1256. doi: 10.1016/j.neuron.2016.04.041. Epub 2016 May 26. Neuron. 2016. PMID: 27238865
-
Poznanski RR. Poznanski RR. J Integr Neurosci. 2005 Sep;4(3):341-61. doi: 10.1142/s0219635205000860. J Integr Neurosci. 2005. PMID: 16178062 Review.
-
Famiglietti EV Jr. Famiglietti EV Jr. Vision Res. 1983;23(11):1265-79. doi: 10.1016/0042-6989(83)90102-5. Vision Res. 1983. PMID: 6362185 Review.
Cited by
-
Ezra-Tsur E, Amsalem O, Ankri L, Patil P, Segev I, Rivlin-Etzion M. Ezra-Tsur E, et al. PLoS Comput Biol. 2021 Dec 30;17(12):e1009754. doi: 10.1371/journal.pcbi.1009754. eCollection 2021 Dec. PLoS Comput Biol. 2021. PMID: 34968385 Free PMC article.
-
Wang B, Zhang Y. Wang B, et al. PLoS Biol. 2023 Sep 18;21(9):e3002301. doi: 10.1371/journal.pbio.3002301. eCollection 2023 Sep. PLoS Biol. 2023. PMID: 37721959 Free PMC article.
-
El-Quessny M, Feller MB. El-Quessny M, et al. eNeuro. 2021 Sep 7;8(5):ENEURO.0261-21.2021. doi: 10.1523/ENEURO.0261-21.2021. Print 2021 Sep-Oct. eNeuro. 2021. PMID: 34380656 Free PMC article.
-
Preserving inhibition with a disinhibitory microcircuit in the retina.
Chen Q, Smith RG, Huang X, Wei W. Chen Q, et al. Elife. 2020 Dec 3;9:e62618. doi: 10.7554/eLife.62618. Elife. 2020. PMID: 33269700 Free PMC article.
-
On the potential role of lateral connectivity in retinal anticipation.
Souihel S, Cessac B. Souihel S, et al. J Math Neurosci. 2021 Jan 9;11(1):3. doi: 10.1186/s13408-020-00101-z. J Math Neurosci. 2021. PMID: 33420903 Free PMC article.
References
Publication types
MeSH terms
Substances
LinkOut - more resources
Full Text Sources
Other Literature Sources
Molecular Biology Databases
Miscellaneous