CD93 promotes β1 integrin activation and fibronectin fibrillogenesis during tumor angiogenesis - PubMed
- ️Mon Jan 01 2018
. 2018 Aug 1;128(8):3280-3297.
doi: 10.1172/JCI97459. Epub 2018 Jun 25.
Affiliations
- PMID: 29763414
- PMCID: PMC6063507
- DOI: 10.1172/JCI97459
CD93 promotes β1 integrin activation and fibronectin fibrillogenesis during tumor angiogenesis
Roberta Lugano et al. J Clin Invest. 2018.
Abstract
Tumor angiogenesis occurs through regulation of genes that orchestrate endothelial sprouting and vessel maturation, including deposition of a vessel-associated extracellular matrix. CD93 is a transmembrane receptor that is upregulated in tumor vessels in many cancers, including high-grade glioma. Here, we demonstrate that CD93 regulates β1 integrin signaling and organization of fibronectin fibrillogenesis during tumor vascularization. In endothelial cells and mouse retina, CD93 was found to be expressed in endothelial filopodia and to promote filopodia formation. The CD93 localization to endothelial filopodia was stabilized by interaction with multimerin-2 (MMRN2), which inhibited its proteolytic cleavage. The CD93-MMRN2 complex was required for activation of β1 integrin, phosphorylation of focal adhesion kinase (FAK), and fibronectin fibrillogenesis in endothelial cells. Consequently, tumor vessels in gliomas implanted orthotopically in CD93-deficient mice showed diminished activation of β1 integrin and lacked organization of fibronectin into fibrillar structures. These findings demonstrate a key role of CD93 in vascular maturation and organization of the extracellular matrix in tumors, identifying it as a potential target for therapy.
Keywords: Brain cancer; Fibronectin; Oncology; Vascular Biology; endothelial cells.
Conflict of interest statement
Conflict of interest: The authors have declared that no conflict of interest exists.
Figures
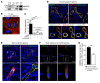
(A) Western blot against MMRN2 in CD93 coimmunoprecipitated samples (CD93 co-IP) derived from total protein lysates of HDMECs. A nonrelevant IgG antibody was used as negative control (IgG co-IP). CD93 FT and IgG FT represent the flow-through of the unbound fraction. (B) In situ proximity ligation assay (PLA) for CD93 and MMRN2 in cultured HDBECs. Positive signal (green dots) indicates proximity between CD93 and MMRN2. F-actin was stained by phalloidin (red) and nuclei by Hoechst (blue). Scale bar: 20 μm. (C) Quantification of CD93 and MMRN2 interaction based on the number of positive signals per cell (n = 3 independent experiments). Nonspecific signals (PLA negative controls) were also examined. **P < 0.01; 1-way ANOVA with Dunnett’s multiple-comparisons test. (D–F) Immunofluorescent staining of MMRN2, CD93, and CD31 in human grade IV glioma vessels (D), in orthotopic GL261 glioma vasculature (E), and in nontumor brain vasculature adjacent to a GL261 tumor (F). Scale bars in all pictures: 20 μm. (G) MMRN2 quantification in tumor and nontumor vessels of WT (n = 3) and CD93–/– (n = 3) mice. Values represent mean ± SEM expressed as arbitrary units (AU) of MMRN2-positive area normalized by CD31-positive area. **P < 0.01; 2-tailed t test.
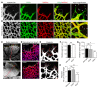
(A) CD93 and MMRN2 immunofluorescent staining in the sprouting front of P6 WT mouse retina. The vasculature is visualized by isolectin B4. Scale bars: 20 μm. High-magnification picture shows CD93 localized in the filopodia and CD93/MMRN2 colocalization in the sprouting front. Arrowheads indicate secreted MMRN2 residing in the extracellular matrix close to the filopodia. (B) CD93 and MMRN2 staining in the retinal plexus region. High magnification shows CD93/MMRN2 colocalization in the vasculature. Arrowheads indicate secreted MMRN2. Scale bars: 20 μm. (C) Radial expansion of vessels in WT and CD93–/– mouse retina. The red line indicates the extension of the vascular network. Scale bars: 150 μm. (D) Vascular sprouts at the front of the retinal vasculature in WT and CD93–/– mice. The length of the endothelial sprouting front is indicated by a dotted line. Nuclei were visualized by ERG (blue). Scale bars: 50 μm. (E) Filopodia protrusions in WT and CD93–/– mice. Red dots indicate filopodia in the sprouting front. Scale bars: 20 μm. (F) Quantification of radial expansion in WT (n = 14) and CD93–/– (n = 7) mice. Values indicate the vessel migration length expressed in micrometers. Values represent mean ± SEM; 2-tailed t test. (G) Quantification of the sprouting front length in WT (n = 7), CD93+/– (n = 4), and CD93–/– (n = 7) mice. Values indicate the length of the sprouts relative to the migrating front length. (H) Filopodia quantification in WT (n = 5), CD93+/– (n = 4), and CD93–/– (n = 5) mice. Values indicate the number of filopodia per 100 μm vessel length. *P < 0.05, **P < 0.01; 1-way ANOVA with Dunnett’s multiple-comparisons test.
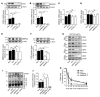
(A) Western blot and real-time quantitative PCR (qPCR) showing the efficiency of CD93 knockdown in HDBECs (siCD93_1 and siCD93_2) at 48 hours after siRNA transfection. Untransfected cells (Mock) and scramble siRNA-transfected cells (siCtrl) were used as controls. (B) Western blot and real-time qPCR for MMRN2 showing the efficiency of MMRN2 knockdown in HDBECs (siMMRN2_3 and siMMRN2_6). (C) MMRN2 mRNA levels after CD93 knockdown in HDBECs. (D) CD93 mRNA level after MMRN2 knockdown. Values represent fold change relative to siCtrl-transfected cells. (E) Western blot against MMRN2 in siCD93-transfected cells and controls. β-Actin was used as loading control. (F) Western blot against CD93 and β-actin in siMMRN2-transfected cells and controls. (G) Western blot against CD93 in control and siMMRN2-silenced HDBECs in the presence or absence of 50 μg/ml cycloheximide (CHX). The stability of CD93 in control cells and in siMMRN2-silenced cells was assessed at different time points after CHX treatment (0, 30, 60, and 240 minutes). (H) Quantification of CD93 levels during specific time points in the presence of 50 μg/ml CHX in control cells and siMMRN2-silenced cells. Values represent the percentage of CD93 relative to CHX-untreated cells in all conditions (control and siMMRN2 cells). *P < 0.05; 2-way ANOVA with Tukey’s post-test. Values represent mean ± SEM (3 independent experiments). (I) Representative Western blot of soluble CD93 (sCD93) levels in conditioned media supplemented with 1%FBS (EBM 1%FBS) derived from control and siMMRN2-silenced cells. (J) Quantification of sCD93 levels in the conditioned media derived from control and siMMRN2-treated cells. Values shown in the quantification graphs in A–F and J represent mean ± SEM (3 independent experiments); *P < 0.05, **P < 0.001; 1-way ANOVA with Dunnett’s multiple-comparisons test.
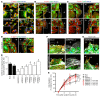
(A) Immunofluorescent staining of CD93 (green), phalloidin (red), and nuclei (blue) in control (Mock and siCtrl) and MMRN2-silenced (siMMRN2_3 and siMMRN2_6) HDBECs cultured under nonconfluent conditions. High-magnification images show localization of CD93 in the filopodia. Scale bars: 20 μm. (B) CD93 (green) in the migrating front of control and siMMRN2-silenced cells at 6 hours after production of a double-sided scratch in the cell monolayer. Arrows indicate direction of migration. High-magnification pictures show CD93 localization in the migrating front of control and siMMRN2 cells. Scale bars: 20 μm. (C and D) Rescue experiment performed using a 2-well culture insert enabling coculturing of control cells and siMMRN2-transfected cells during migration. Immunofluorescent staining of CD93 (green), phalloidin (red), and nuclei (blue). Scale bars: 20 μm. CD93 localization was assessed after 6 hours of migration in control and siMMRN2 cells (C) and siMMRN2 cells cocultured with control cells (D). (E) Quantification of CD93 in the migrating front area. Bars represent arbitrary units (AU) of the CD93-positive signal at the migrating front area depicted by dotted line in C and D. *P < 0.05 vs. Mock, #P < 0.05 vs. siCtrl, §vs. siMMRN2_3, ‡vs. siMMRN2_6; 1-way ANOVA with Dunnett’s post-test. Values represent mean ± SEM (4 different areas along the migrating edge in 3 independent experiments). (F and G) Costaining of CD93 (green), MMRN2 (red), and phalloidin (gray) in the migrating front of control and siMMRN2 cells and siMMRN2 cells cultured during 24 hours in conditioned medium derived from control cells (+siCtrl CM). Scale bars: 20 μm. (H) Migration assay in control cells, MMRN2-downregulated cells, and siMMRN2-transfected cells cultured in control conditioned medium (CM). Values represent mean ± SEM (3 independent experiments); *P < 0.05, **P < 0.01; 1-way ANOVA with Dunnett’s multiple-comparisons test.
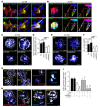
(A) Immunofluorescent staining of CD93 and MMRN2 in the sprouting front (indicated by dotted line) of vessels formed in embryoid bodies (EBs) cultured in 2D on glass slides upon VEGF treatment. Differentiated endothelial cells were visualized by CD31 staining. Scale bars: 20 μm. Specific localization of CD93 and MMRN2 in CD31-positive sprouts and filopodia is shown in high-magnification images. (B) Immunofluorescent staining of CD93 and MMRN2 in EBs cultured in a 3D collagen gel. Scale bars: 200 μm. High-magnification images show a specific localization of CD93 in the tip cells and in filopodia. MMRN2 staining indicates its colocalization with CD31-positive sprouts and with CD93 in the tip cells. (C) CD31 immunofluorescent staining of shCD93- and shMMRN2-transfected EBs cultured in 2D glass slide. Untransfected (Ctrl) or empty vector–transfected (shCtrl) EBs were used as controls. Scale bars: 500 μm. (D) Quantification of CD31 sprouting expansion. Values represent mean ± SEM (at least n = 5 EBs per condition). ***P < 0.001; 1-way ANOVA with Dunnett’s multiple-comparisons test. (E) CD31 immunofluorescent staining of shCD93- and shMMRN2-transfected or control EBs (Ctrl and shCtrl) cultured in 3D collagen gel. Scale bars: 500 μm. (F) Quantification of CD31-positive sprouts. Values represent mean ± SEM (at least n = 10 EBs per condition). **P < 0.01, ***P < 0.001; 1-way ANOVA with Dunnett’s multiple-comparisons test. (G) Coculture of 2 individual EBs of each condition in 2D glass slide. (H) Coculture of control EBs (Ctrl or shCtrl) with shMMRN2 or shCD93 EBs. (I) Quantification of the CD31 sprouting expansion rescued upon coculture of control EBs with shMMRN2 or shCD93 EBs. *P < 0.05 vs. Ctrl; #P < 0.05 vs. shCtrl; 1-way ANOVA with Dunnett’s multiple-comparisons test.
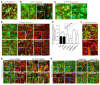
(A) CD93 (green) and MMRN2 (red) colocalization in confluent HDBECs. High-magnification picture shows CD93-MMRN2 colocalizing in fibrillary structures. Scale bar: 20 μm. (B) CD93 (green) and fibronectin (red) costaining in control or MMRN2-silenced (siMMRN2_3) confluent HDBECs. Scale bars: 20 μm. (C) MMRN2 (green) and fibronectin (red) costaining in control or CD93-downregulated cells (siCD93_1). (D) Immunofluorescent staining of fibronectin (green) and F-actin (red) in control, siCD93-treated, and siMMRN2-treated HDBECs cultured under confluent conditions on gelatin-coated surfaces. Scale bars: 20 μm. (E) Real-time qPCR for fibronectin in control (Mock and siCtrl), CD93-downregulated (siCD93_1 and siCD93_2), and MMRN2-silenced cells (siMMRN2_3 and siMMRN2_6). Values represent mean ± SEM (3 independent experiments); 1-way ANOVA with Dunnett’s multiple-comparisons test. (F) Fibronectin (green) and F-actin (red) in brain endothelial cells isolated from WT and CD93–/– mice and cultured on gelatin-coated surfaces. Scale bars: 20 μm. (G) Rescue of fibronectin fibrillogenesis disrupted by CD93 downregulation. Immunofluorescent staining for fibronectin (green) and F-actin (red) in HDMECs stably expressing WT-CD93 insensitive to siRNA knockdown (WT-CD93 HDMEC) or untransfected cells (Control HDMEC) after endogenous CD93 silencing (siCD93_1 and siCD93_2). Scale bars: 20 μm. (H) Rescue of fibronectin fibrillogenesis upon MMRN2 downregulation (siMMRN2) in the presence or absence of conditioned medium derived from control cells (+Mock CM or +siCtrl CM). Immunofluorescent staining for fibronectin (green) and F-actin (red). Scale bars: 20 μm.
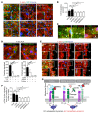
(A) Immunofluorescent staining of the active β1 integrin (12G10) in control, CD93-silenced (siCD93_1, siCD93_2), and MMRN2-silenced (siMMRN2_3, siMMRN2_6) HDBECs cultured under confluent conditions. Scale bars: 20 μm. (B) Quantification of 12G10 staining in control, siCD93, and siMMRN2 cells. Values represent mean ± SEM expressed as arbitrary units (AU) of 12G10-positive area normalized by cell number (n = 8 different areas of the cell monolayer). *P < 0.05. (C) Colocalization between CD93 and α5β1 integrin and active β1 (12G10) is shown by arrowheads. Different distribution of CD93 and αvβ3 integrin is shown by arrowheads. Scale bars: 20 μm. (D) In situ PLA for CD93 and α5β1 integrin as well as CD93 and active β1 integrin (12G10) in HDBECs. Positive signal (green dots) indicates proximity between CD93 and integrins. F-actin (red) and nuclei (blue). Scale bars: 20 μm. Bars represent the quantification of CD93 and α5β1 integrin or CD93 and active β1 integrin interaction based on the number of positive signals per cell (n = 3 independent experiments). *P < 0.05; **P < 0.01. (E) Y397-phosphorylated FAK staining (p-FAK) in the migrating front of control or siCD93- and siMMRN2-silenced cells at 6 hours after scratch wound. Arrows indicate the direction of migration. Scale bars: 20 μm. (F) Quantification of the p-FAK–positive signal along the migrating front (20 μm distance from the migrating front, indicated by dotted line in E). Bars represent arbitrary units (AU) of p-FAK signal normalized by the number of cells at the migrating edge. *P < 0.05. Values represent mean ± SEM (4 different areas along the migrating edge, 3 independent experiments). 1-Way ANOVA with Dunnett’s post-test was used in all graphs. (G) Schematic representation of CD93 and MMRN2 interacting complex. FB, fibronectin; MMRN2, multimerin-2; sCD93, soluble CD93; Int α5β1, α5β1 integrin; p-FAK, phosphorylated focal adhesion kinase.
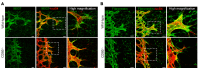
(A) Immunofluorescent staining of active β1 integrin (9EG7) in WT and CD93–/– P6 mouse retina. The vasculature is visualized by isolectin B4. Scale bars: 20 μm. High-magnification images show inhibition of active β1 integrin in the filopodia of CD93–/– compared with WT mouse retina. (B) Immunofluorescent staining of fibronectin and isolectin B4 in WT and CD93–/– P6 mouse retina. Scale bars: 20 μm. High-magnification image shows a disruption of the fibronectin matrix in the proximity of endothelium and filopodia in CD93–/– compared with WT mouse retina.
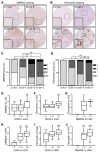
(A and B) Immunohistochemical staining of MMRN2 (A) and fibronectin (B) in nontumor control brain and WHO grade II, grade III, and grade IV human glioma tissue. (C and D) Semiquantitative scoring of the fraction of MMRN2-positive (C) and fibronectin-positive (D) blood vessels in human glioma tissue microarrays. The frequency of positively staining vessels in 235 samples from human glioma and nontumor control brain was scored in a blinded fashion on a scale from 0 to 3 (0, no vessels stained; 1, minority of vessels stained; 2, majority of vessels stained; 3 strong staining in the majority of vessels). Results were averaged and plotted as percentage of samples per score in nontumor control brain (2 biopsies), WHO grade II (65 biopsies), grade III (29 biopsies), and grade IV samples (69 biopsies); *P < 0.005, **P < 0.0001; 1-way ANOVA with Tukey’s post-test. (E–G) Comparison between scoring of MMRN2 and CD93 (E), of fibronectin and CD93 (F), and of fibronectin and MMRN2 (G) in low-grade glioma (LGG, WHO grade II). *P < 0.05 vs. <1 score; #P < 0.05 vs. 1–2 score; 1-way ANOVA with Tukey’s post-test. Comparison between scoring of MMRN2 and CD93 (H), of fibronectin and CD93 (I), and of fibronectin and MMRN2 (J) in high-grade glioma (HGG, WHO grade III–IV). *P < 0.05, **P < 0.005, ***P < 0.0001 vs. <1 score; 1-way ANOVA with Tukey’s post-test.
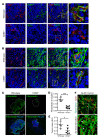
(A) Immunofluorescent staining of the active conformation of β1 integrin (9EG7) in GL261 tumor vasculature of WT and CD93–/– mice. CD31 was used as vascular marker (red) and Hoechst as nuclear marker (blue). High-magnification images show the levels of 9EG7 staining associated with the vasculature in WT and CD93–/– mice. Scale bars: 20 μm. (B) Immunofluorescent staining of fibronectin and CD31 in GL261 tumor vasculature of WT and CD93–/– mice. High-magnification images show fibronectin associated with the vasculature in WT and CD93–/– mice. Scale bars: 20 μm. (C) Tile scan of GL261 tumor in WT and CD93–/– mice stained for fibronectin. Scale bars: 500 μm. (D) Quantification of fibronectin-positive signal in WT (n = 4) and CD93–/– (n = 6) mice. Values represent mean ± SEM expressed as arbitrary units (AU) of fibronectin-positive area normalized by total tumor area. ***P < 0.0001; 2-tailed t test. (E) Quantification of tumor area in WT (n = 4) and CD93–/– (n = 6) mice. Values represent mean ± SEM expressed as AU of the total tumor area determined by nuclei staining. *P < 0.05; 2-tailed t test. (F) 3D volume reconstruction of fibronectin (green) and CD31 (red) immunofluorescent staining in a GL261 vibratome section. Arrowheads indicate fibronectin fibers associated with vessels (CD31 positive). Scale bar: 20 μm.
Similar articles
-
Khan KA, Naylor AJ, Khan A, Noy PJ, Mambretti M, Lodhia P, Athwal J, Korzystka A, Buckley CD, Willcox BE, Mohammed F, Bicknell R. Khan KA, et al. Oncogene. 2017 Nov 2;36(44):6097-6108. doi: 10.1038/onc.2017.214. Epub 2017 Jul 3. Oncogene. 2017. PMID: 28671670 Free PMC article.
-
Galvagni F, Nardi F, Spiga O, Trezza A, Tarticchio G, Pellicani R, Andreuzzi E, Caldi E, Toti P, Tosi GM, Santucci A, Iozzo RV, Mongiat M, Orlandini M. Galvagni F, et al. Matrix Biol. 2017 Dec;64:112-127. doi: 10.1016/j.matbio.2017.08.003. Epub 2017 Sep 11. Matrix Biol. 2017. PMID: 28912033
-
Langenkamp E, Zhang L, Lugano R, Huang H, Elhassan TE, Georganaki M, Bazzar W, Lööf J, Trendelenburg G, Essand M, Pontén F, Smits A, Dimberg A. Langenkamp E, et al. Cancer Res. 2015 Nov 1;75(21):4504-16. doi: 10.1158/0008-5472.CAN-14-3636. Epub 2015 Sep 11. Cancer Res. 2015. PMID: 26363010
-
C-type lectin domain group 14 proteins in vascular biology, cancer and inflammation.
Khan KA, McMurray JL, Mohammed F, Bicknell R. Khan KA, et al. FEBS J. 2019 Sep;286(17):3299-3332. doi: 10.1111/febs.14985. Epub 2019 Jul 29. FEBS J. 2019. PMID: 31287944 Free PMC article. Review.
-
Galectin-8: a matricellular lectin with key roles in angiogenesis.
Troncoso MF, Ferragut F, Bacigalupo ML, Cárdenas Delgado VM, Nugnes LG, Gentilini L, Laderach D, Wolfenstein-Todel C, Compagno D, Rabinovich GA, Elola MT. Troncoso MF, et al. Glycobiology. 2014 Oct;24(10):907-14. doi: 10.1093/glycob/cwu054. Epub 2014 Jun 16. Glycobiology. 2014. PMID: 24939370 Review.
Cited by
-
Wu B, Fu L, Guo X, Hu H, Li Y, Shi Y, Zhang Y, Han S, Lv C, Tian Y. Wu B, et al. Front Immunol. 2023 Jan 25;14:984816. doi: 10.3389/fimmu.2023.984816. eCollection 2023. Front Immunol. 2023. PMID: 36761750 Free PMC article.
-
Yu X, Zhang H, Li J, Gu L, Cao L, Gong J, Xie P, Xu J. Yu X, et al. Front Cell Dev Biol. 2024 Feb 5;12:1237445. doi: 10.3389/fcell.2024.1237445. eCollection 2024. Front Cell Dev Biol. 2024. PMID: 38374893 Free PMC article.
-
Loss of Multimerin-2 and EMILIN-2 Expression in Gastric Cancer Associate with Altered Angiogenesis.
Andreuzzi E, Capuano A, Pellicani R, Poletto E, Doliana R, Maiero S, Fornasarig M, Magris R, Colombatti A, Cannizzaro R, Spessotto P, Mongiat M. Andreuzzi E, et al. Int J Mol Sci. 2018 Dec 11;19(12):3983. doi: 10.3390/ijms19123983. Int J Mol Sci. 2018. PMID: 30544909 Free PMC article.
-
Zhang Z, Zheng M, Ding Q, Liu M. Zhang Z, et al. Front Cell Dev Biol. 2022 Feb 15;10:817965. doi: 10.3389/fcell.2022.817965. eCollection 2022. Front Cell Dev Biol. 2022. PMID: 35242761 Free PMC article.
-
CD93 Signaling via Rho Proteins Drives Cytoskeletal Remodeling in Spreading Endothelial Cells.
Barbera S, Raucci L, Lugano R, Tosi GM, Dimberg A, Santucci A, Galvagni F, Orlandini M. Barbera S, et al. Int J Mol Sci. 2021 Nov 17;22(22):12417. doi: 10.3390/ijms222212417. Int J Mol Sci. 2021. PMID: 34830297 Free PMC article.
References
Publication types
MeSH terms
Substances
LinkOut - more resources
Full Text Sources
Other Literature Sources
Miscellaneous