Evolutionary History of Bacteriophages in the Genus Paraburkholderia - PubMed
- ️Mon Jan 01 2018
Evolutionary History of Bacteriophages in the Genus Paraburkholderia
Akbar Adjie Pratama et al. Front Microbiol. 2018.
Abstract
The genus Paraburkholderia encompasses mostly environmental isolates with diverse predicted lifestyles. Genome analyses have shown that bacteriophages form a considerable portion of some Paraburkholderia genomes. Here, we analyzed the evolutionary history of prophages across all Paraburkholderia spp. Specifically, we investigated to what extent the presence of prophages and their distribution affect the diversity/diversification of Paraburkholderia spp., as well as to what extent phages coevolved with their respective hosts. Particular attention was given to the presence of CRISPR-Cas arrays as a reflection of past interactions with phages. We thus analyzed 36 genomes of Paraburkholderia spp., including those of 11 new strains, next to those of three Burkholderia species. Most genomes were found to contain at least one full prophage sequence. The highest number was found in Paraburkholderia sp. strain MF2-27; the nine prophages found amount to up to 4% of its genome. Among all prophages, potential moron genes (e.g., DNA adenine methylase) were found that might be advantageous for host cell fitness. Co-phylogenetic analyses indicated the existence of complex evolutionary scenarios between the different Paraburkholderia hosts and their prophages, including short-term co-speciation, duplication, host-switching and phage loss events. Analysis of the CRISPR-Cas systems showed a record of diverse, potentially recent, phage infections. We conclude that, overall, different phages have interacted in diverse ways with their Paraburkholderia hosts over evolutionary time.
Keywords: Paraburkholderia; evolution; mobile genetic elements; prophages; soil.
Figures
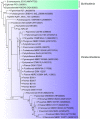
Phylogeny of the 36 Paraburkholderia and three Burkholderia species used in this study. The 16S rRNA genes of the 39 bacteria were aligned using the SINA (Pruesse et al., 2007) and MAFFT (Katoh et al., 2002) alignment tools. The alignment was edited using Gblocks (Talavera et al., 2007). A maximum likelihood based phylogenetic tree was then constructed using FastTree (Price et al., 2009) and the tree (midpoint-rooting) was visualized using iTOL (Letunic and Bork, 2016). Gray circles represent “plant-associated” Paraburkholderia species, while white circles represent “non-plant-associated” Paraburkholderia species. See section Materials and Methods for explanation of plant- vs. non-plant-association.
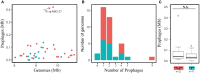
Prophage distribution. (A) Prophage size depicted as related to host genome size. Dots in blue correspond to plant-associated Paraburkholderia spp., while red dots correspond to non-plant-associated Paraburkholderia spp. *Represents the outlier Paraburkholderia sp. MF2-27. (B) Distribution of the number of prophages per genome. (C) Box-plot of the distribution of size of prophage genomes (Mb) of plant-associated and non-plant-associated Paraburkholderia spp. (Kolmogorov-Smirnov test, N.S.–not significant, ρ = 0.3454). See section Materials and Methods for explanation of “P”–plant- vs. “N-P” non-plant-association.
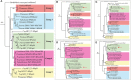
Complete prophage relatedness trees based on (A) concatenated phage signature (capsid, portal, tape, and terminase) genes and individual phage genes, i.e., (B) capsid, (C) portal, (D) tape, and (E) terminase. The translations of concatenated and individual signature genes were aligned with MUSCLE (Edgar, 2004) and edited using Gblocks (Talavera et al., 2007). Maximum likelihood phylogenetic trees were constructed using FastTree (Price et al., 2009) and trees (midpoint-rooting) were visualized using iTOL (Letunic and Bork, 2016). Colors represent consistent groupings of the prophages. Red arrows indicate phage ϕ437.
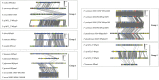
Synteny analysis of complete prophage genomes. The groupings of the prophages are based on the phylogenetic tree constructed with the concatenated phage signature (i.e., capsid, portal, tape, and terminase) genes. Red arrows: phage lysis and lysogeny genes; blue arrows: phage structural genes (tail, capsid, and fiber); green arrows: replication, recombination, repressor, and phage related genes; gray arrows: hypothetical proteins; yellow arrows: non-phage or possible moron genes. Comparison percentage was generated using BLAST + 2.4.0 (tBLASTx with cutoff value 10−3) and map comparison figures were created with Easyfig (Sullivan et al., 2011) as indicated in section Materials and Methods. Gene similarity percentage is indicated in gray-scale bars.
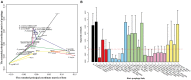
PACo (Procrustes approach to co-phylogeny) results based on Paraburkholderia species phylogeny and concatenated prophage phylogeny. (A) Procrustean superimposition plot analysis, which minimizes differences between the two partners' principal correspondence coordinates of patristic distances. For each vector, the starting point (black dot) represents the configuration of prophages and the arrowhead the configuration of hosts. The vector length represents the global fit (residual sum of squares), which is inversely proportional to the topological congruence. (B) Contribution of each Paraburkholderia lineage and their prophages to the general pattern of coevolution. Each bar represents a Jack-knifed squared residual. Error bars represent the upper 95% confidence intervals from applying PACo to patristic distances. Further, the median squared residual value is shown (dashed red line). The colors represent the clusters shown in the prophage phylogeny (see Figure 3).
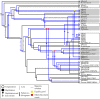
Tanglegram depicting the Paraburkholderia (host) species phylogenetic tree in black and the prophage tree in blue. Auxiliary lines connecting the two trees are shown. The event-based method was performed with the default settings for cost regimes (“co-speciation” event = 0; “duplication” event = 1; “loss” event = 1; “duplication then host switching” event = 2) using Jane 4.0 (Conow et al., 2010). All analyses were performed with populations of 1,000 and 10 generations. Gray boxes represent plant-associated Paraburkholderia species and white boxes non-plant-associated Paraburkholderia species. Jane results showed that host-switching events occurred frequently, next to co-speciation.
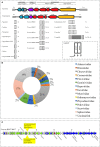
(A) CRISPR-Cas systems identified in Paraburkholderia species. The Cas genes are colored according to their functional category, as in Makarova et al. (2015). CRISPR arrays are represented as black boxes, with black lines representing repeats and white filling denoting spacers. Numbers on top of the CRISPR arrays represent the number of CRISPR-spacers. (B) BLAST-based hits of spacer sequences and (C) Yersinia pekkanenii CIP110230 and Y. pekkanenii A125KOH2 proto-spacer hits, analyzed using IMG/VR (Paez-Espino et al., 2017).
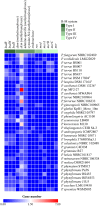
Heat map representing restriction-modification (R-M) systems found in the Paraburkholderia species. The rows represent the genomes tested for the presence of genes in R-M systems. The horizontal axis represents gene numbers in R-M systems. The color scale represents the number of genes, with dark blue squares representing the absence of matches and red squares representing the highest numbers of matches.
Similar articles
-
A novel inducible prophage from the mycosphere inhabitant Paraburkholderia terrae BS437.
Pratama AA, van Elsas JD. Pratama AA, et al. Sci Rep. 2017 Aug 22;7(1):9156. doi: 10.1038/s41598-017-09317-8. Sci Rep. 2017. PMID: 28831124 Free PMC article.
-
Prediction of Prophages and Their Host Ranges in Pathogenic and Commensal Neisseria Species.
Orazi G, Collins AJ, Whitaker RJ. Orazi G, et al. mSystems. 2022 Jun 28;7(3):e0008322. doi: 10.1128/msystems.00083-22. Epub 2022 Apr 14. mSystems. 2022. PMID: 35418239 Free PMC article.
-
Wang G, Liu Q, Pei Z, Wang L, Tian P, Liu Z, Zhao J, Zhang H, Chen W. Wang G, et al. Front Microbiol. 2020 May 26;11:1088. doi: 10.3389/fmicb.2020.01088. eCollection 2020. Front Microbiol. 2020. PMID: 32528454 Free PMC article.
-
Comparative genomics of phages and prophages in lactic acid bacteria.
Desiere F, Lucchini S, Canchaya C, Ventura M, Brüssow H. Desiere F, et al. Antonie Van Leeuwenhoek. 2002 Aug;82(1-4):73-91. Antonie Van Leeuwenhoek. 2002. PMID: 12369206 Review.
-
Research progress of prophages.
Chen X, Wei Y, Ji X. Chen X, et al. Yi Chuan. 2021 Mar 16;43(3):240-248. doi: 10.16288/j.yczz.20-355. Yi Chuan. 2021. PMID: 33724208 Review.
Cited by
-
Flament-Simon SC, de Toro M, Chuprikova L, Blanco M, Moreno-González J, Salas M, Blanco J, Redrejo-Rodríguez M. Flament-Simon SC, et al. Sci Rep. 2020 Jul 27;10(1):12452. doi: 10.1038/s41598-020-69356-6. Sci Rep. 2020. PMID: 32719405 Free PMC article.
-
Use of Specific Borrelia Phages as a New Strategy for Improved Diagnostic Tests.
Shan J, Jia Y, Mijatovic T. Shan J, et al. Methods Mol Biol. 2024;2742:99-104. doi: 10.1007/978-1-0716-3561-2_8. Methods Mol Biol. 2024. PMID: 38165618
-
Weiser R, Yap ZL, Otter A, Jones BV, Salvage J, Parkhill J, Mahenthiralingam E. Weiser R, et al. Viruses. 2020 May 31;12(6):601. doi: 10.3390/v12060601. Viruses. 2020. PMID: 32486377 Free PMC article.
-
Cryptic Prophages Contribution for Campylobacter jejuni and Campylobacter coli Introgression.
Tanoeiro L, Oleastro M, Nunes A, Marques AT, Duarte SV, Gomes JP, Matos APA, Vítor JMB, Vale FF. Tanoeiro L, et al. Microorganisms. 2022 Feb 26;10(3):516. doi: 10.3390/microorganisms10030516. Microorganisms. 2022. PMID: 35336092 Free PMC article.
-
Klonowska A, Ardley J, Moulin L, Zandberg J, Patrel D, Gollagher M, Marinova D, Reddy TBK, Varghese N, Huntemann M, Woyke T, Seshadri R, Ivanova N, Kyrpides N, Reeve W. Klonowska A, et al. Front Microbiol. 2023 Feb 28;14:1082107. doi: 10.3389/fmicb.2023.1082107. eCollection 2023. Front Microbiol. 2023. PMID: 36925474 Free PMC article.
References
LinkOut - more resources
Full Text Sources
Other Literature Sources
Miscellaneous