Shared evolutionary origin of vertebrate neural crest and cranial placodes - PubMed
Shared evolutionary origin of vertebrate neural crest and cranial placodes
Ryoko Horie et al. Nature. 2018 Aug.
Abstract
Placodes and neural crests represent defining features of vertebrates, yet their relationship remains unclear despite extensive investigation1-3. Here we use a combination of lineage tracing, gene disruption and single-cell RNA-sequencing assays to explore the properties of the lateral plate ectoderm of the proto-vertebrate, Ciona intestinalis. There are notable parallels between the patterning of the lateral plate in Ciona and the compartmentalization of the neural plate ectoderm in vertebrates4. Both systems exhibit sequential patterns of Six1/2, Pax3/7 and Msxb expression that depend on a network of interlocking regulatory interactions4. In Ciona, this compartmentalization network produces distinct but related types of sensory cells that share similarities with derivatives of both cranial placodes and the neural crest in vertebrates. Simple genetic disruptions result in the conversion of one sensory cell type into another. We focused on bipolar tail neurons, because they arise from the tail regions of the lateral plate and possess properties of the dorsal root ganglia, a derivative of the neural crest in vertebrates5. Notably, bipolar tail neurons were readily transformed into palp sensory cells, a proto-placodal sensory cell type that arises from the anterior-most regions of the lateral plate in the Ciona tadpole6. Proof of transformation was confirmed by whole-embryo single-cell RNA-sequencing assays. These findings suggest that compartmentalization of the lateral plate ectoderm preceded the advent of vertebrates, and served as a common source for the evolution of both cranial placodes and neural crest3,4.
Figures
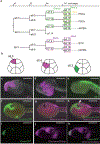
a, Cell lineages of anterior blastomeres (a-line blastomeres) and a posterior blastomere (b-line) from the 16-cell to 110-cell stages. Green identifies Dmrt.a-expressing blastomeres, magenta identifies Msxb expression lineages, and yellow identifies Foxc expression. b, Schematic illustration of 16 cell stage embryos. Each of the blastomeres that was labeled with Dil or DiO is indicated by magenta or green. c-e, Head regions of larvae labeled with DiI or DiO at the 16 cell stage. c, Labeling of the a5.3 lineage. d, Labeling of the a5.4 lineage. e, labeling b5.3 blastomere. f-h, Head region of a larva that was co-injected with Dmrt.a>CFP and Msxb>mCherry reporter genes. Arrowhead identifies the boundary of the Dmrt.a/Msxb expression territories. i-k, Head region of a larva co-injected with Foxc>CFP and Dmrt.a>mCherry reporter genes. Anterior to the left; scale bars, 100μm.
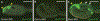
a-c, Head regions of larvae injected with an Eya>CFP reporter gene. Yellow arrowheads denote Eya expression in the proto-placodal region of Control MO injected larvae (36 of 36 larvae displayed this pattern) (a). c, there is expanded expression (white arrowheads) in Msxb morphants (39 of 48 larvae showed this phenotype). b, there is a loss of expression in Dmrt.a morphants (88 of 88 larvae showed this phenotype). Anterior to the left; scale bars, 100μm.
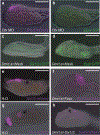
a, b, Head regions of larvae that were injected with an Otx MO, and co-injected with Six1/2>mCherry (a) and Eya>CFP (b) (32 of 32 larvae showed reduced or no expression of Six1/2>mCherry and no expression of Eya>CFP). c, d, Head regions of larvae injected with Dmrt.a>Msxb, and co-injected with Six1/2>CFP (33 of 33 larvae showed no expression of Six1/2>CFP) (c) and Eya>CFP (71 of 71 larvae showed little or no expression of Eya>CFP) (d). e, f, Head regions of larvae injected with Six1/2>mCherry, and co-injected with H2O (61 of 61 larvae showed full expression of Six1/2>mCherry) (e), or Dmrt.a>Foxc (50 of 50 larvae showed no expression of Six1/2>mCherry) (f). g, h, Head regions of larvae injected with Foxc>mCherry, and co-injected with H2O (40 of 40 larvae showed full expression of Foxc>mCherry (g) or Dmrt.a>Six1/2 (39 of 39 larvae showed no expression of Foxc>mCherry) (h). Scale bars, 100μm.
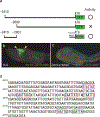
a Deletion analyses of the 5’ regulatory region of Six1/2. −2410 bp to −2001 bp of the 5’ cis-regulatory region is necessary for Kaede expression in the pre-placodal territory. b, c, head regions of larvae injected with Six1/2 −2410/−2001>Kaede, and co-injected with H2O (74 of 74 larvae showed full expression of Six1/2 −2410/−2001>Kaede) or Dmrt.a>Msxb (37 of 37 larvae showed no expression of Six1/2 2410/−2001>Kaede) (c). d, the Six1/2 5’ regulatory region spanning −2410 to −2001 bp contains an Otx binding site (green box) and multiple Msxb repressor binding sites (magenta box).
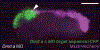
Tailbud embryo co-injected with Dmrt.a MO, Dmrt.a Δ MO target sequence>CFP and Msxb>mCherry construct. The anterior expansion of the Msxb>mCherry expression pattern is indicated by the white arrowhead. Anterior to the left; scale bars, 100μm

a-c, Larvae injected with CNGA>CFP reporter gene. Yellow arrowheads indicate expression in aATENs, and white arrowheads indicate ectopic sites of differentiated aATENs in the palp. a, Larva co-injected with Dmrt.a MO (62 of 62 larvae showed no expression of CNGA>CFP in aATENs). b, Larva co-injected with control MO (32 of 32 larvae showed full expression of CNGA>CFP). c, Larva co-injected with Foxc MO (18 of 42 larvae showed expanded expression of CNGA>CFP in palp region). Anterior to the left; scale bars, 100μm.
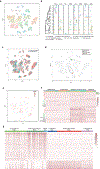
a, b, Identification of major cell types among 20 clusters with known markers, 3 epidermis clusters were identified by the expression of EpiB; 2 clusters of epidermis and sensory neurons were identified by the expression of either Msxb or Nut, along with EpiB; a cluster of sensory neurons identified by the expression of NGN3 and Nut; 3 clusters of central nervous system by high levels of Nut transcripts; 6 clusters of mesenchyme by their expression of Twist; 2 endoderm clusters by the high expression of SOD3; and one notochord cluster by the expression of Ci-T (Brachyury). c, Representation of overlap between cells from Pax3/7>Foxc and control embryos in each cell population. tSNE plot from Figure 3a, with each cell now colored to indicate their origin from either Pax3/7>Foxc embryos (red dots, n=5339) or control embryos (blue dots, n=4850). Both samples contribute to all 20 cell populations. d, Identification of BTNs and PSCs with the combination of representation markers in the control embryo, 27 BTNs (green dot) were identified by the combination of Asic1b & Synaphin, and 15 PSCs were identified by the combination of Foxg, islet, and SP8. e, Visualization of SV40 positive cells in Pax3/7>Foxc transgenic embryos within tSNE projection map. SV40 is detected in cells contained within clusters 2 and 5 for epidermis and sensory neuron cells, as well as weak expression in mesenchyme clusters 15 and 16. None of the transformed or hybrid cells contained in the sensory cell clusters (5 and 6) express any mesenchyme marker genes, suggesting that none of these are transformed by misexpression of Pax3/7. f, heatmap of representative genes (see Fig. 3e of main text) that show no significant differential expression in SV40 positive cells contained within clusters 2, 5, 15, and 16. g, heatmap of all differentially expressed genes between BTNs and PSCs from both control and Pax3/7>Foxc embryos.
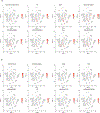
Distribution of new identified marker gene in PSCs (a) and BTNs (b).
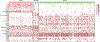
Transcription factors (red), signaling pathway genes (green), and effector genes (black).
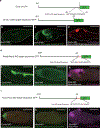
a-d, Dmrt.a MO. a, Schematic diagram of reporter gene containing Dmrt.a regulatory genes with and without recognition sequences for the Dmrt.a MO that was used in this study. The MO recognition sequences are located in the 5’ UTR, upstream of the initiating AUG codon (−1). b, Larva injected with Dmrt.a MO and co-injected with the Dmrt.a>CFP reporter gene containing the MO recognition sequences. 46 of 46 larvae showed no protein synthesis from the Dmrt.a>CFP reporter. c, Same as b except that the reporter gene lacks the Dmrt.a MO recognition sequence (Dmrt.a ΔMO target>CFP). 57 of 57 larvae showed CFP expression in appropriate head tissues. Dmrt.a MO efficiently blocks the expression of CFP that contains the Dmrt.a MO target sequence. d, Larva co-injected with Dmrt.a MO, Dmrt.a ΔMO>Dmrt.a and Six1/2>mCherry. Dmrt.a MO morphants normally lack Six1/2>mCherry expression (see Fig. 2b), but expression is restored with a Dmrt.a transgene that lacks the MO recognition sequence (107 of 108 larvae showed expression of Six1/2>mCherry). This result shows that the Dmrt.a MO used in this study specifically blocks the synthesis of Dmrt.a protein products. e-h, Msxb MO. e, Diagram of Msxb 5’ regulatory region and the location of the recognition sequences for the Msxb MO and point mutations in this sequence. f, g, Larvae injected with Msxb> CFP containing MO recognition sequences, and co-injected with control MO (54/54 larvae showed CFP expression) (f). g, Same as f except that the Msxb MO was coinjected in place of the control MO (44/44 larvae showed no expression of CFP). These results show that the Msxb MO specifically blocks CFP protein synthesis from the Msxb reporter gene. h, Larva co-injected with Msxb MO, Six1/2>mCherry, and Msxb>CFP reporter gene containing point mutations in MO recognition sequence (see red letters in panel e). Msxb morphants normally display expanded expression of Six1/2 in tail regions (see Figure 2c). This expansion is suppressed by co-injection of the mutant Msxb transgene lacking MO recognition sequences (h). This result suggests that the Msxb MO inhibits synthesis of Msxb protein products. i-l, Foxc MO. i, Diagram of Foxc 5’ regulatory region showing the location of the MO recognition sequence and point mutations in this sequence. j-l, Larvae injected with Foxc>Foxc transgene and Foxc>CFP reporter gene, and also co-injected with control MO (25/25 larvae showed CFP expression) (j). k, Same as j except that the embryo was co-injected with the Foxc MO in place of the control MO (99/99 larvae showed no expression of CFP) (k). This result shows that the Foxc MO efficiently blocks the synthesis of CFP proteins encoded by the Foxc>CFP reporter gene containing the Foxc MO target sequence. l, Larvae co-injected with Foxc MO, Foxc>Foxc mut (MO-resistant Foxc cDNA) and βγ-crystallin>mCherry. Normally, Foxc morphants lack expression of the βγ-crystallin>mCherry reporter gene (see Figure 2f). However, expression is restored by co-injection of Foxc>Foxc transgene. This result suggests that the Foxc MO inhibits synthesis of Foxc protein products. Scale bar, 100μm.
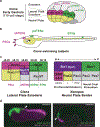
a, Summary of lateral plate derivatives at early gastrula (110 cell stage). Magenta indicates progenitors of palp sensory cells (PSCs) and aATENs arising from the a8.20, a8.18 and a8.26 lineages, respectively. Green indicates pATENs and BTNs arising from b8.20 and b8.18, respectively. b, Diagram of Ciona tadpole showing the position of PSCs, aATENs, pATENs and BTNs. c, Summary of Ciona lateral plate ectoderm and Xenopus pan-placodal primordium. Magenta indicates Dmrt.a expressing blastomeres (a8.20, a8.18 and a8.26 lineage), green indicates Msxb expressing blastomeres (b8.20 and b8.18 lineage), violet indicates prospective Six1/2 and Eya expressing blastomeres (a8.26 lineage), yellow indicates Foxc expressing blastomeres (a8.20 and a8.18 lineage) and grey indicates Pax3/7 expressing blastomeres (a8.26, b8.20 and b8.18 lineage), A, anterior; Ad, adenohypophyseal placode; OL, olfactory placode; L, lens placode; P, posterior; Pr, profundal placode; V, trigeminal placode. d, (left) Tailbud embryo co-injected with Dmrt.a>CFP (green) and Msxb>mCherry (magenta) reporter genes. Arrowhead indicates the boundary separating the Dmrt.a and Msxb expression territories. d, (right) Tailbud embryo injected with Six1/2>CFP and Foxc>mCherry reporter genes. Arrowhead indicates the boundary separating the Six1/2 and Foxc expression territories. Anterior to the left; scale bars, 100 μm.
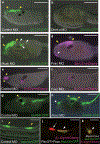
a-c, Head regions of larvae that were injected with a Six1/2>CFP reporter gene. a, Six1/2 expression in the proto-placodal region of control MO injected tadpoles (49 of 49 larvae displayed this expression pattern). The yellow arrowheads identify the normal location of Six1/2 expression. b, Loss of expression in Dmrt.a morphants (49 of 49 larvae). c, Expanded expression of the Six1/2>CFP reporter gene (white arrowheads) in Msxb morphants (42 of 50 larvae showed this expansion pattern). d, Head regions of a larva that was co-injected with Foxc MO and Six1/2>mCherry reporter gene. There is ectopic expression (white arrowhead) in the palp regions of Foxc morphants (35 of 47 larvae showed this phenotype). e, f, Larvae injected with βγ-crystallin>mCherry reporter gene. Yellow arrowheads indicate the βγ-crystallin expressing PSCs in control MO injected larvae (51 of 51 larvae display this expression pattern). f, There is a loss of these cells in Foxc morphants (108 of 108 larvae showed this phenotype). g, h, Larvae injected with a GnRH>CFP reporter gene. Yellow arrowheads identify the GnRH expressing aATENs in a control larva (59 of 59 larvae displayed expression in aATENs). h, There is ectopic expression in the palp regions of Foxc morphants (white arrow) (28 of 40 injected larvae showed this phenotype). i-k, Tail regions of larvae injected with Asic1b>CFP (i) and co-injected with βγ-crystallin>mCherry reporter gene (j,k). Yellow arrowheads identify the Asic1b expressing BTNs in a control larva (83 of 83 larvae displayed this phenotype). j, Ectopic expression of the βγ-crystallin>mCherry reporter gene in tail regions (white arrowheads) upon misexpression of Foxc by the Pax3/7 enhancer (26 of 55 larvae showed this phenotype. k, Same as j except that Msxb regulatory sequences were used to misexpress Foxc (31 of 57 larvae showed misexpression of βγ-crystallin>mCherry. Anterior to the left; scale bars, 100 μm (a-h), 20 μm (i-k).
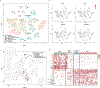
a, The tSNE projection map of dissociated cells from wild-type and mutant tailbud-stage embryos that misexpress Foxc in tail regions using Pax3/7 regulatory DNAs (Pax3/7>Foxc transgene). Each dot corresponds to the transcriptome of a single cell, and cells possessing similar transcriptome profiles map near each other. All of the major tissue types in tailbud-stage embryos were identified: Ep=epidermis; Se=sensory neurons; CNS=central nervous system; Me=mesenchyme; M&H=heart and muscle; En=Endoderm; Noto=Notochord). Identification is based on the expression of known marker genes (Extended Data Fig. 8b, and Extended File 1 sheet 1). b, Distribution of marker gene expressed in PSCs (Islet, Foxg and SP8) and BTNs (Asic1b and Synaphin) within tSNE projections as shown in (a). d, Distribution of cells expressing transgenes, which identifies cells that misexpress the PSC determinant, Foxc. BTNs (dark green dots; n = 21), PSCs (red dots; n=32), hybrid cells that express both PSC and BTN marker genes (light blue triangles; n=14), and transformed cells that express PSC markers (blue dots; n=10). The grey dots (n = 10,103) correspond to all dissociated cells that were sequenced in these experiments. d, Heatmap of BTNs, PSCs, transformed cells, and hybrid cells showing the relative expression of a select group of genes encoding transcription factors (red), signaling components (green), and cellular effectors (black).
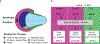
a, Schematic illustration of the neural plate and its lateral border in vertebrate (top half) and ascidians (bottom half). We proposed that both proto-placode and proto-neural crest evolved from entire lateral plate in the last shared tunicate/vertebrate ancestor. Otolith/ocellus contribution to the proto-neural crest is based on Abitua et al., 2012. b, Provisional gene regulatory network for the compartmentalization, specification and differentiation of the lateral plate ectoderm into akin sensory cell types in Ciona intestinalis. During early embryogenesis, Foxc and Six1/2 determine the head /trunk boundary (white dotted line) of proto-placode lineages (magenta) while Msxb patterns the proto-neural crest territory (green). In tailbud stages, a common developmental program specifies sensory progenitors within each compartment. CNGA, RXFP3 and SOG/Chemokine-like expression is based on Abitua et al., 2015.
Similar articles
-
The evolutionary history of vertebrate cranial placodes II. Evolution of ectodermal patterning.
Schlosser G, Patthey C, Shimeld SM. Schlosser G, et al. Dev Biol. 2014 May 1;389(1):98-119. doi: 10.1016/j.ydbio.2014.01.019. Epub 2014 Feb 1. Dev Biol. 2014. PMID: 24491817 Review.
-
Do vertebrate neural crest and cranial placodes have a common evolutionary origin?
Schlosser G. Schlosser G. Bioessays. 2008 Jul;30(7):659-72. doi: 10.1002/bies.20775. Bioessays. 2008. PMID: 18536035
-
A new model for olfactory placode development.
Whitlock KE. Whitlock KE. Brain Behav Evol. 2004;64(3):126-40. doi: 10.1159/000079742. Brain Behav Evol. 2004. PMID: 15353905 Review.
-
Establishing the pre-placodal region and breaking it into placodes with distinct identities.
Saint-Jeannet JP, Moody SA. Saint-Jeannet JP, et al. Dev Biol. 2014 May 1;389(1):13-27. doi: 10.1016/j.ydbio.2014.02.011. Epub 2014 Feb 24. Dev Biol. 2014. PMID: 24576539 Free PMC article. Review.
-
Vertebrate cranial placodes as evolutionary innovations--the ancestor's tale.
Schlosser G. Schlosser G. Curr Top Dev Biol. 2015;111:235-300. doi: 10.1016/bs.ctdb.2014.11.008. Epub 2015 Jan 20. Curr Top Dev Biol. 2015. PMID: 25662263 Review.
Cited by
-
Pax3/7 regulates neural tube closure and patterning in a non-vertebrate chordate.
Kim K, Orvis J, Stolfi A. Kim K, et al. Front Cell Dev Biol. 2022 Sep 12;10:999511. doi: 10.3389/fcell.2022.999511. eCollection 2022. Front Cell Dev Biol. 2022. PMID: 36172287 Free PMC article.
-
Ishida T, Satou Y. Ishida T, et al. Nat Ecol Evol. 2024 Jun;8(6):1154-1164. doi: 10.1038/s41559-024-02387-8. Epub 2024 Apr 2. Nat Ecol Evol. 2024. PMID: 38565680
-
Shaping faces: genetic and epigenetic control of craniofacial morphogenesis.
Selleri L, Rijli FM. Selleri L, et al. Nat Rev Genet. 2023 Sep;24(9):610-626. doi: 10.1038/s41576-023-00594-w. Epub 2023 Apr 24. Nat Rev Genet. 2023. PMID: 37095271 Review.
-
Johnson CJ, Razy-Krajka F, Stolfi A. Johnson CJ, et al. Evodevo. 2020 Aug 3;11:15. doi: 10.1186/s13227-020-00162-x. eCollection 2020. Evodevo. 2020. PMID: 32774829 Free PMC article.
-
The regulation of oocyte maturation and ovulation in the closest sister group of vertebrates.
Matsubara S, Shiraishi A, Osugi T, Kawada T, Satake H. Matsubara S, et al. Elife. 2019 Oct 1;8:e49062. doi: 10.7554/eLife.49062. Elife. 2019. PMID: 31573508 Free PMC article.
References
-
- Northcutt RG & Gans C The genesis of neural crest and epidermal placodes: a reinterpretation of vertebrate origins. Q. Rev. Biol 58, 1–28 (1983). - PubMed
-
- Baker CV & Bronner-Fraser M The origins of the neural crest. Part II: an evolutionary perspective. Mech. Dev 69, 13–29 (1997). - PubMed
-
- Schlosser G Do vertebrate neural crest and cranial placodes have a common evolutionary origin? Bioessays 30, 659–672 (2008). - PubMed
-
- Schlosser G, Patthey C & Shimeld SM The evolutionary history of vertebrate cranial placodes II. Evolution of ectodermal patterning. Dev. Biol 389, 98–119 (2014). - PubMed
Publication types
MeSH terms
Substances
LinkOut - more resources
Full Text Sources
Other Literature Sources
Molecular Biology Databases
Research Materials