Tree frog attachment: mechanisms, challenges, and perspectives - PubMed
- ️Mon Jan 01 2018
Review
Tree frog attachment: mechanisms, challenges, and perspectives
Julian K A Langowski et al. Front Zool. 2018.
Abstract
Tree frogs have the remarkable ability to attach to smooth, rough, dry, and wet surfaces using their versatile toe pads. Tree frog attachment involves the secretion of mucus into the pad-substrate gap, requiring adaptations towards mucus drainage and pad lubrication. Here, we present an overview of tree frog attachment, with focus on (i) the morphology and material of the toe pad; (ii) the functional demands on the toe pad arising from ecology, lifestyle, and phylogenetics; (iii) experimental data of attachment performance such as adhesion and friction forces; and (iv) potential perspectives on future developments in the field. By revisiting reported data and observations, we discuss the involved mechanisms of attachment and propose new hypotheses for further research. Among others, we address the following questions: Do capillary and hydrodynamic forces explain the strong friction of the toe pads directly, or indirectly by promoting dry attachment mechanisms? If friction primarily relies on van der Waals (vdW) forces instead, how much do these forces contribute to adhesion in the wet environment tree frogs live in and what role does the mucus play? We show that both pad morphology and measured attachment performance suggest the coaction of several attachment mechanisms (e.g. capillary and hydrodynamic adhesion, mechanical interlocking, and vdW forces) with situation-dependent relative importance. Current analytical models of capillary and hydrodynamic adhesion, caused by the secreted mucus and by environmental liquids, do not capture the contributions of these mechanisms in a comprehensive and accurate way. We argue that the soft pad material and a hierarchical surface pattern on the ventral pad surface enhance the effective contact area and facilitate gap-closure by macro- to nanoscopic drainage of interstitial liquids, which may give rise to a significant contribution of vdW interactions to tree frog attachment. Increasing the comprehension of the complex mechanism of tree frog attachment contributes to a better understanding of other biological attachment systems (e.g. in geckos and insects) and is expected to stimulate the development of a wide array of bioinspired adhesive applications.
Keywords: Attachment organ; Bioadhesion; Biomimetics; Biotribology; Capillary adhesion; Drainage; Litoria caerulea; Lubrication; Toe pad; van der Waals.
Conflict of interest statement
Not applicable.Not applicable.The authors declare that they have no competing interests.Springer Nature remains neutral with regard to jurisdictional claims in published maps and institutional affiliations.
Figures
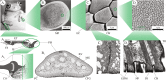
Morphology of a digit and toe pad of a hylid tree frog. A Macroscopic structures. (A 1) Forelimbs of Litoria caerulea. (A 2) Schematic lateral view of tendons, phalangi (dark grey), and the intercalary element (light grey) in a digit of Scinax squalirostris. (A 3) Schematic depiction of the collagen fibres in a pad of Hyla dominicensis in dorsal view. B Superficial and internal pad structures in L. caerulea. (B 1) SEM image of the ventral epidermis. (B 2) Transverse section through the toe of a juvenile frog. C Epidermal cells on the ventral surface. (C 1) SEM image of polygonal cells in L. caerulea. (C 2) TEM image of a tangential cross-section through the apical part of two adjacent cells in Hyla cinerea. D Fine structures of the apical surface of an epidermal cell. (D 1) High power SEM image of nanopillars and their central depressions (‘dimples’) in L. caerulea. (D 2) TEM image of a cross-section through a row of nanopillars in H. cinerea (black arrows: EDM). BV blood vessels, CFG circumferential groove, CH channel between two epidermal cells/nanopillars, CO collagen fibres, D dermis, DE dorsal epidermis, DI dimple, EC epidermal cell, EDM electron dense material, ET extensor brevis profundus tendon, IE intercalary element, MG mucus gland, MP mucus pore, NP nanopillar, PC pad curvature, PH (terminal) phalanx, TO tonofilaments, TS tendo superficialis, VE ventral epidermis. The illustrations are not to scale. A 1, B 1, C 1 and D 1 modified after [25]; A 2 modified after [66]; A 3 modified after [44]; B 2 modified after [47]; C 2 and D 1 modified after [23]. All figures printed with permission
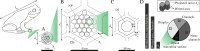
Geometrical model of the ventral toe pad epidermis in a tree frog with snout-vent-length ℓSV. A The approximately circular projected ventral pad area Ap (diameter dp) is B covered by polygonal epidermal cells with diameter dc, edge length ac, channel width wc, and apical surface area Ac. C Each cell accommodates polygonal nanopillars with diameter dn, channel width wn, and a distance Δr between dimple and nanopillar edge. D Enhancement (in %) of the wetted area by the micro- and nanochannels relative to Ap and composition of Ap (in %) assuming regular, hexagonal outlines of the epidermal cells and nanopillars. Inset: Definition of projected and wetted areas shown for a hexagonal pillar. CH channel, DI dimple, NP nanopillar
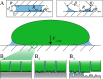
A Schematic representation of capillary adhesion between a toe pad (green) and a hydrophilic substrate caused by the formation of a mucus meniscus (blue). Left inset: Capillary adhesion between two flat, solid plates. Right inset: Capillary adhesion between a solid sphere and a flat, solid plate. B Hypothesised changes in wetting state with an increase in substrate roughness or pad-substrate gap width [97]. dg gap width, F⊥,cap capillary adhesion, R sphere radius, Rmer, Razi meridional and azimuthal radius of meniscus curvature, β filling angle, γ mucus surface tension, ϕ contact angle. B modified after [97]. Printed with permission
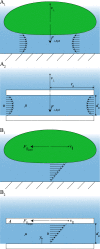
Hydrodynamic (A) adhesion and (B) friction (1) during the schematic interaction between a toe pad (green) and the substrate based on displacement-induced flow of mucus (blue) and (2) in a model of the contact of two flat and rigid (cylindrical) plates. A area, dg gap width, F⊥,hyd hydrodynamic adhesion, F∥,hyd hydrodynamic friction, rp plate radius, u flow speed, v⊥ detachment speed, v∥ sliding speed, y spatial coordinate normal to the substrate, μ viscosity
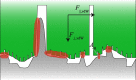
Schematic generation of van der Waals (vdW) forces (F⊥,vdW, F∥,vdW) between ventral toe pad epidermis (green) and substrate (grey) for a system-specific Hamaker constant AH. VdW interactions occur in regions of close pad-substrate contact (red)
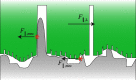
Schematic mechanical interlocking between a superficial cell (left star) or a nanopillar (right star) on the ventral toe pad epidermis (green) and asperities of a rough substrate (grey) at a shear load F∥,L
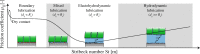
Stribeck diagram showing the proposed spectrum of lubrication modes and the resulting friction coefficients μ∥ in tree frogs’ toe pads as a function of the Stribeck number St

Hypothesised regimes of mucus drainage in a tree frog’s toe pad. A Far field regime. B Intermediate field regime. C Near field regime. D Drainage through the nanochannel network and E the nanopillar-substrate gap. DI dimple, EC epidermal cell, NP nanopillar. dg gap width, u flow speed. Modified after [99]. Printed with permission
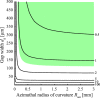
Tenacity contours [mN mm −2] computed for capillary adhesion at varying gap widths (i.e. twice the meridional radius of meniscus curvature) and azimuthal radii of meniscus curvature (≈0.5 dp), respectively, according to Eq. 1. We assumed ϕ= 0° and γ= 71.97 mN m–1. The green patch shows the combinations of Razi and Rmer that lead to tenacities in the range of measured values [47]
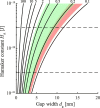
Tenacity contours [mN mm −2] computed for van der Waals (vdW) interactions at various Hamaker constants and gap widths according to Equation 7. We assumed that 10% of the contact area contributes to vdW force generation. Dashed lines: Theoretical minimum Hamaker constant for water separating two similar materials at 26 °C (bottom) and a 10-fold higher Hamaker constant (top). Coloured patches show the combinations of AH and dg leading to tenacities in the range of measured values ([47], green; [69], red)
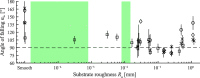
Variation of the falling angle α⊥ with substrate roughness Ra on an inclined, dry substrate (circles from Fig. 5 in [43], Hyla microcephala; crosses from Fig. 8A in [9], Colostethus trinitatis; diamonds from Fig. 3B in [10], Rhacophorus pardalis; squares from Fig. 1B in [97], Litoria caerulea). Green areas denote the diameter range of nanopillars (left) and epidermal cells (right) reported in the main text. Dashed line: Falling angles below 90° show the lack of adhesive abilities. Most roughness values mentioned in the references are approximations and do not originate from measurements
Similar articles
-
Langowski JKA, Schipper H, Blij A, van den Berg FT, Gussekloo SWS, van Leeuwen JL. Langowski JKA, et al. J Anat. 2018 Oct;233(4):478-495. doi: 10.1111/joa.12860. Epub 2018 Aug 19. J Anat. 2018. PMID: 30123974 Free PMC article.
-
Design of Tree-Frog-Inspired Adhesives.
Langowski JKA, Dodou D, van Assenbergh P, van Leeuwen JL. Langowski JKA, et al. Integr Comp Biol. 2020 Oct 1;60(4):906-918. doi: 10.1093/icb/icaa037. Integr Comp Biol. 2020. PMID: 32413122 Free PMC article. Review.
-
Wet but not slippery: Boundary friction in tree frog adhesive toe pads.
Federle W, Barnes WJ, Baumgartner W, Drechsler P, Smith JM. Federle W, et al. J R Soc Interface. 2006 Oct 22;3(10):689-97. doi: 10.1098/rsif.2006.0135. J R Soc Interface. 2006. PMID: 16971337 Free PMC article.
-
Estimating the maximum attachment performance of tree frogs on rough substrates.
Langowski JKA, Rummenie A, Pieters RPM, Kovalev A, Gorb SN, van Leeuwen JL. Langowski JKA, et al. Bioinspir Biomim. 2019 Feb 1;14(2):025001. doi: 10.1088/1748-3190/aafc37. Bioinspir Biomim. 2019. PMID: 30706849
-
Meng F, Liu Q, Wang X, Tan D, Xue L, Barnes WJP. Meng F, et al. Philos Trans A Math Phys Eng Sci. 2019 Jul 29;377(2150):20190131. doi: 10.1098/rsta.2019.0131. Epub 2019 Jun 10. Philos Trans A Math Phys Eng Sci. 2019. PMID: 31177956 Free PMC article. Review.
Cited by
-
Bio-macromolecular design roadmap towards tough bioadhesives.
Montazerian H, Davoodi E, Baidya A, Badv M, Haghniaz R, Dalili A, Milani AS, Hoorfar M, Annabi N, Khademhosseini A, Weiss PS. Montazerian H, et al. Chem Soc Rev. 2022 Oct 31;51(21):9127-9173. doi: 10.1039/d2cs00618a. Chem Soc Rev. 2022. PMID: 36269075 Free PMC article. Review.
-
Functional adhesive hydrogels for biological interfaces.
Liu C, Peng K, Wu Y, Fu F. Liu C, et al. Smart Med. 2023 Oct 7;2(4):e20230024. doi: 10.1002/SMMD.20230024. eCollection 2023 Nov. Smart Med. 2023. PMID: 39188302 Free PMC article. Review.
-
Bio-inspired adhesive hydrogel for biomedicine-principles and design strategies.
Li W, Yang X, Lai P, Shang L. Li W, et al. Smart Med. 2022 Dec 25;1(1):e20220024. doi: 10.1002/SMMD.20220024. eCollection 2022 Dec. Smart Med. 2022. PMID: 39188733 Free PMC article. Review.
-
Chudak M, Chopra V, Hensel R, Darhuber AA. Chudak M, et al. Langmuir. 2020 Oct 13;36(40):11929-11937. doi: 10.1021/acs.langmuir.0c02005. Epub 2020 Oct 2. Langmuir. 2020. PMID: 32903008 Free PMC article.
-
Borodich FM, Gao Z, Gorb EV, Gorb SN, Jin X. Borodich FM, et al. Biomimetics (Basel). 2024 Jul 19;9(7):442. doi: 10.3390/biomimetics9070442. Biomimetics (Basel). 2024. PMID: 39056883 Free PMC article. Review.
References
-
- Peattie AM. Functional demands of dynamic biological adhesion: an integrative approach. J Comp Physiol B. 2009;179:231–9. - PubMed
-
- Gorb SN. Biological attachment devices: exploring nature’s diversity for biomimetics. Phil Trans R Soc A. 2008;366:1557–74. - PubMed
-
- Niewiarowski PH, Stark AY, Dhinojwala A. Sticking to the story: outstanding challenges in gecko-inspired adhesives. J Exp Biol. 2016;219(7):912–9. - PubMed
-
- Gorb S, editor. Attachment Structures and, Adhesive Secretions in Arachnids. vol. 7. Cham, Switzerland: Springer; 2016.
Publication types
LinkOut - more resources
Full Text Sources
Other Literature Sources
Miscellaneous