Heavy Metal Neurotoxicants Induce ALS-Linked TDP-43 Pathology - PubMed
- ️Tue Jan 01 2019
Heavy Metal Neurotoxicants Induce ALS-Linked TDP-43 Pathology
Peter E A Ash et al. Toxicol Sci. 2019.
Abstract
Heavy metals, such as lead, mercury, and selenium, have been epidemiologically linked with a risk of ALS, but a molecular mechanism proving the connection has not been shown. A screen of putative developmental neurotoxins demonstrated that heavy metals (lead, mercury, and tin) trigger accumulation of TDP-43 into nuclear granules with concomitant loss of diffuse nuclear TDP-43. Lead (Pb) and methyl mercury (MeHg) disrupt the homeostasis of TDP-43 in neurons, resulting in increased levels of transcript and increased splicing activity of TDP-43. TDP-43 homeostasis is tightly regulated, and positively or negatively altering its splicing-suppressive activity has been shown to be deleterious to neurons. These changes are associated with the liquid-liquid phase separation of TDP-43 into nuclear bodies. We show that lead directly facilitates phase separation of TDP-43 in a dose-dependent manner in vitro, possibly explaining the means by which lead treatment results in neuronal nuclear granules. Metal toxicants also triggered the accumulation of insoluble TDP-43 in cultured cells and in the cortices of exposed mice. These results provide novel evidence of a direct mechanistic link between heavy metals, which are a commonly cited environmental risk of ALS, and molecular changes in TDP-43, the primary pathological protein accumulating in ALS.
Figures
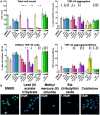
A screen of neurotoxins in inducible stably expressing cells reveals heavy metals trigger formation of nuclear TDP-43:: GFP inclusions. PC12 cells expressing TDP-43:: GFP were screened against developmental neurotoxins in the NTP HTS NeuroTox 91 Plate (Chemical list, Supplementary Table 1). High-content imaging was used to quantify cell count, number of cells with diffuse TDP-43:: GFP and number of cells with nuclear TDP-43:: GFP inclusions (full screening results in Supplementary Figs. 1A–E). A, Four compounds were found to induce formation of TDP-43 inclusions: lead (II) acetate trihydrate (compound ID: F2), methyl mercuric (II) chloride (F5, H5), colchicine (D8) (concentrations: 1.56, 6.25, 25, and 100 µM) and Bis(tributyltin)oxide (C10) (concentrations: 0.1, 0.39, 1.56, and 6.25 µM). N = 4; mean ±SEM; ANOVA w/Dunnett’s multiple comparison test, *p < .05, **p < .01, ***p < .01. B, Representative images of merged GFP and DAPI signals show cells with diffuse nuclear TDP-43:: GFP upon DMSO (vehicle control) treatment and nuclear TDP-43:: GFP inclusions upon exposure to lead (II) acetate trihydrate, methyl mercuric (II) chloride, bis(tributyltin)oxide, and colchicine (at concentrations shown). Representative images of positive control (2 µM arsenite), uninduced PC12 cells, and different compound doses are shown in Supplementary Figure 2.
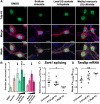
Exposure to lead and methyl mercury triggers formation of neuronal nuclear inclusions of endogenous Tdp-43. A, Primary hippocampal neurons (DIV14) exposed for 6 h to lead (II) acetate trihydrate and methyl mercuric (II) chloride develop nuclear inclusions of endogenous Tdp-43. Neurons were immunolabelled with antibodies to MAP2 (magenta) and Tdp-43 (green) and nuclei were counterstained with DAPI (blue). Shown are representative images of neurons treated with 2 µM sodium arsenite, 4.69 µM lead (II) acetate trihydrate, and 1.56 µM methyl mercuric (II) chloride. Secondary only controls show no immunolabel (Supplementary Figure 3). A masking algorithm (Imaris, Bitplane) was used to detect and quantify inclusions per neuronal nuclear. B, Lead (II) acetate trihydrate concentrations: 0.174, 0.521, 1.56, and 4.69 µM; methyl mercuric (II) chloride concentrations: 0.058, 0.174, 0.521, and 1.56 µM. N = 4; mean ± SEM; ANOVA w/Dunnett’s multiple comparison test versus DMSO control, *p < 0.05, **p < 0.01, ***p < 0.01. C, Lead at 1.56 µM effects the alternative splicing function of Tdp-43 leading to reduced ratio of inclusion of Sort1 exon 17b compared with wild-type Sort1 transcripts. This negatively correlates (Supplementary Figure 4E; slope = −0.65 ± 0.21, R2 = 0.39, p = .0074) with an increase in transcript levels of Tdp-43 (Tardbp) (D) upon lead treatment. N = 6; mean ±SEM; ANOVA w/Dunnett’s multiple comparison test, *p < .05, **p < .01.
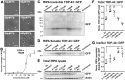
Lead triggers phase separation of TDP-43 and decreases its solubility. A, Purified TDP-43 LLPS in vitro is facilitated by lead (II) acetate trihydrate (Pb) in a dose-dependent manner. Representative 63× DIC images. Arrows: examples of single TDP-43 droplets. Asterisk: examples of amorphous TDP-43 consolidates. Arrowheads: high-contrast, inert 1 micron polystyrene microspheres added to aid sample focusing. Scale bar = 10 µm. B, LLPS was quantified using an ImageJ algorithm as percentage of ROI covered by droplets. Points at mean, with error bars at SEM, were fit by nonlinear regression analysis (line) and the LogEC50 calculated as 160 µM (dotted line). Induced PC12 cells accumulate insoluble TDP-43:: GFP upon treatment with lead (Pb). Immunoblots of RIPA insoluble (C), RIPA soluble (D) and total RIPA lysate (E) were probed with anti-GFP antibodies (N = 3). F, Densitometric analysis of total TDP-43:: GFP/Actin bands (from panel E) show an increase in TDP-43:: GFP in response to 0.174 and 0.521 µM lead but an decrease in response to 4.69 µM lead. G, At 1.56 and 4.69 µM concentrations, lead significantly increases the ratio of insoluble TDP-43:: GFP to soluble TDP-43:: GFP. N = 3; mean ± SEM; ANOVA w/Dunnett’s multiple comparison test, *p < .05, **p < .01. AU: arbitrary units. Predicted molecular weight of TDP-43:: GFP ∼70 kDa. Uninduced PC12 cells express no TDP-43:: GFP.
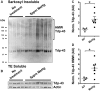
Methyl mercury exposure results in cortical accumulation of insoluble Tdp-43. Cortical tissue from mice exposed, by ingestion, to 0 ppm (no treatment, N = 4) and 5 ppm methyl mercuric (II) chloride (MeHg, N = 7) was fractionated by solubility. The sarkosyl insoluble (A) and total lysate (B) (TE: Tris-EDTA) fractions were immunoblotted and probed for endogenous Tdp-43. Upon systemic MeHg exposure, sarkosyl insoluble Tdp-43 (C) and high molecular weight (HMW) Tdp-43 (D) is significantly increased. Mean ±SEM; unpaired two-tailed t test, *p < .05. AU: arbitrary units.
Similar articles
-
Wang C, Duan Y, Duan G, Wang Q, Zhang K, Deng X, Qian B, Gu J, Ma Z, Zhang S, Guo L, Liu C, Fang Y. Wang C, et al. Mol Cell. 2020 Aug 6;79(3):443-458.e7. doi: 10.1016/j.molcel.2020.06.019. Epub 2020 Jul 9. Mol Cell. 2020. PMID: 32649883
-
Inorganic mercury within motor neurons does not cause the TDP-43 changes seen in sporadic ALS.
Pamphlett R, Kum Jew S. Pamphlett R, et al. Toxicol Lett. 2011 Feb 25;201(1):58-61. doi: 10.1016/j.toxlet.2010.12.005. Epub 2010 Dec 15. Toxicol Lett. 2011. PMID: 21167262
-
Mitchell JC, Constable R, So E, Vance C, Scotter E, Glover L, Hortobagyi T, Arnold ES, Ling SC, McAlonis M, Da Cruz S, Polymenidou M, Tessarolo L, Cleveland DW, Shaw CE. Mitchell JC, et al. Acta Neuropathol Commun. 2015 Jun 25;3:36. doi: 10.1186/s40478-015-0212-4. Acta Neuropathol Commun. 2015. PMID: 26108367 Free PMC article.
-
Yamashita T, Kwak S. Yamashita T, et al. Brain Res. 2014 Oct 10;1584:28-38. doi: 10.1016/j.brainres.2013.12.011. Epub 2013 Dec 16. Brain Res. 2014. PMID: 24355598 Review.
-
Metals in ALS TDP-43 Pathology.
Koski L, Ronnevi C, Berntsson E, Wärmländer SKTS, Roos PM. Koski L, et al. Int J Mol Sci. 2021 Nov 11;22(22):12193. doi: 10.3390/ijms222212193. Int J Mol Sci. 2021. PMID: 34830074 Free PMC article. Review.
Cited by
-
The amyotrophic lateral sclerosis exposome: recent advances and future directions.
Goutman SA, Savelieff MG, Jang DG, Hur J, Feldman EL. Goutman SA, et al. Nat Rev Neurol. 2023 Oct;19(10):617-634. doi: 10.1038/s41582-023-00867-2. Epub 2023 Sep 14. Nat Rev Neurol. 2023. PMID: 37709948 Free PMC article. Review.
-
Davis DA, Garamszegi SP, Banack SA, Dooley PD, Coyne TM, McLean DW, Rotstein DS, Mash DC, Cox PA. Davis DA, et al. Toxins (Basel). 2021 Oct 1;13(10):697. doi: 10.3390/toxins13100697. Toxins (Basel). 2021. PMID: 34678990 Free PMC article.
-
Risk factors of amyotrophic lateral sclerosis: a global meta-summary.
Duan QQ, Jiang Z, Su WM, Gu XJ, Wang H, Cheng YF, Cao B, Gao X, Wang Y, Chen YP. Duan QQ, et al. Front Neurosci. 2023 Apr 24;17:1177431. doi: 10.3389/fnins.2023.1177431. eCollection 2023. Front Neurosci. 2023. PMID: 37168926 Free PMC article.
-
Spencer PS, Palmer VS. Spencer PS, et al. Toxics. 2021 Mar 16;9(3):57. doi: 10.3390/toxics9030057. Toxics. 2021. PMID: 33809439 Free PMC article. Review.
-
Modelling amyotrophic lateral sclerosis in rodents.
Todd TW, Petrucelli L. Todd TW, et al. Nat Rev Neurosci. 2022 Apr;23(4):231-251. doi: 10.1038/s41583-022-00564-x. Epub 2022 Mar 8. Nat Rev Neurosci. 2022. PMID: 35260846 Review.
References
-
- Arai T., Hasegawa M., Akiyama H., Ikeda K., Nonaka T., Mori H., Mann D., Tsuchiya K., Yoshida M., Hashizume Y., et al. (2006). TDP-43 is a component of ubiquitin-positive tau-negative inclusions in frontotemporal lobar degeneration and amyotrophic lateral sclerosis. Biochem. Biophys. Res. Commun. 351, 602–611. - PubMed
-
- Aschner M., Ceccatelli S., Daneshian M., Fritsche E., Hasiwa N., Hartung T., Hogberg H. T., Leist M., Li A., Mundi W. R., et al. (2017). Reference compounds for alternative test methods to indicate developmental neurotoxicity (DNT) potential of chemicals: Example lists and criteria for their selection and use. Altex 34, 49–74. - PMC - PubMed
-
- Bal-Price A., Coecke S., Costa L., Crofton K. M., Fritsche E., Goldberg A., Grandjean P., Lein P. J., Li A., Lucchini R., et al. (2012). Advancing the science of developmental neurotoxicity (DNT): Testing for better safety evaluation. ALTEX 29, 202–215. - PubMed
Publication types
MeSH terms
Substances
LinkOut - more resources
Full Text Sources
Medical
Miscellaneous