The search for pain biomarkers in the human brain - PubMed
- ️Mon Jan 01 2018
Review
The search for pain biomarkers in the human brain
André Mouraux et al. Brain. 2018.
Abstract
Non-invasive functional brain imaging is used more than ever to investigate pain in health and disease, with the prospect of finding new means to alleviate pain and improve patient wellbeing. The observation that several brain areas are activated by transient painful stimuli, and that the magnitude of this activity is often graded with pain intensity, has prompted researchers to extract features of brain activity that could serve as biomarkers to measure pain objectively. However, most of the brain responses observed when pain is present can also be observed when pain is absent. For example, similar brain responses can be elicited by salient but non-painful auditory, tactile and visual stimuli, and such responses can even be recorded in patients with congenital analgesia. Thus, as argued in this review, there is still disagreement on the degree to which current measures of brain activity exactly relate to pain. Furthermore, whether more recent analysis techniques can be used to identify distributed patterns of brain activity specific for pain can be only warranted using carefully designed control conditions. On a more general level, the clinical utility of current pain biomarkers derived from human functional neuroimaging appears to be overstated, and evidence for their efficacy in real-life clinical conditions is scarce. Rather than searching for biomarkers of pain perception, several researchers are developing biomarkers to achieve mechanism-based stratification of pain conditions, predict response to medication and offer personalized treatments. Initial results with promising clinical perspectives need to be further tested for replicability and generalizability.
Figures
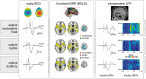
Transient nociceptive stimuli causing pain. In this example heat laser pulses delivered to the right hand (scalp EEG and intracerebral LFP) or foot (functional MRI) elicit large-scale brain responses. In scalp EEG, the response is dominated by a large negative-positive wave maximal at the scalp vertex (electrode Cz), probably originating from bilateral operculo-insular regions, the cingulate cortex and, possibly, the contralateral primary somatosensory cortex. Responses in similar regions are also detected using functional MRI. Importantly, equally salient but non-painful and non-nociceptive tactile or auditory stimuli elicit very similar EEG and functional MRI responses, indicating that most of this activity is unspecific for pain or nociception and, instead, multimodal (Mouraux and Iannetti, 2009; Mouraux et al., 2011). Similarly, although the insula has been proposed to be strongly involved in pain perception, equally salient nociceptive and non-nociceptive stimuli trigger similar local field potentials (LFPs) recorded directly within the insula (Liberati et al., 2016). Nevertheless, other less prominent features of the sampled activity might be more selective for pain or nociception, as reflected by the selective increase of gamma-band oscillations (GBOs) when painful heat stimuli are presented (Liberati et al., 2018). BOLD = blood-oxygen level-dependent; ER% = event-related change in oscillation amplitude; ERP = event-related potential.
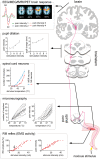
Several physiological measures can, in some circumstances, correlate reliably with the reported intensity of perceived pain. As shown in the left plots, these measures can be obtained at different levels of the neuraxis: peripheral nociceptor activity recorded using microneurography [firing rate of a peripheral C-fibre nociceptor and intensity of perception as a function of stimulation temperature; adapted from Torebjork et al. (1984)], spinal cord activity assessed using dorsal horn electrophysiology in animals [firing rate of WDR and nociceptive specific dorsal horn neuron as a function of stimulation temperature; adapted from Khasabov et al. (2001)] or the recording of nociceptive RIII reflex activity using EMG in humans [amplitude of the RIII nociceptive withdrawal reflex in the tibialis anterior and intensity of perception as a function of the intensity of electric stimulation of the sural nerve; adapted from Willer et al. (1984)], cortical activity sampled using non-invasive functional neuroimaging techniques such as EEG [event-related brain potentials elicited by laser heat stimulation of the hand dorsum as a function of intensity of perception; adapted from Iannetti et al. (2008)], magnetoencephalography (MEG), functional MRI [fMRI-BOLD response elicited by thermal stimulation at different target temperatures; adapted from Bornhovd et al. (2002)] or PET, but also autonomic responses such as pupil dilation [magnitude of pupil dilation and intensity of perception as a function of stimulation intensity; adapted from Chapman et al. (1999)].
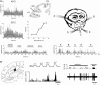
Assessing the selectivity for pain of a given brain response requires not only to demonstrate that the response is present when pain is experienced, but also to demonstrate that it is not present when pain is not experienced. (A) Dong et al. (1994) recorded the activity of a single neuron located in area 7b, close to the secondary somatosensory cortex. When tested with a variety of thermal and mechanical somatic stimuli, the neuron responds to noxious heat stimuli applied to the face in a graded fashion, whereas it does not respond to a variety of mechanical stimuli. Based on these observations one might be tempted to conclude that the neuron is specific for burning pain. However, the same neuron also responds vigorously to visual stimuli approaching its receptive field (A–E), and the response was most prominent when the approaching object was novel or threatening. Therefore, in classical pain studies testing the response properties with noxious and innocuous somatosensory stimuli, this neuron would be labelled as nociceptive-specific (NS). However, this labelling would be incorrect, at least until the lack of responses to a wide range of equally salient, unpleasant and behaviourally-relevant stimuli has been comprehensively demonstrated. (B) Similarly, Hutchison et al. (1999) performed single unit recordings in the human anterior cingulate cortex, and found many neurons responding to noxious heat stimuli, and not to slow-rising innocuous mechanical stimuli. However, some of these neurons also responded to watching noxious stimuli being delivered to the experimenter, suggesting that they may serve a supramodal function related or consequent to stimulus saliency or threat detection. CS = central sulcus; IPS = intraparietal sulcus; LS = lateral sulcus. Adapted with permission from Fig. 7 in Dong et al. (1994) and Fig. 1 in Hutchison et al. (1999).
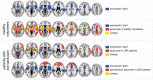
Brain activity related to the perception of acute experimental pain and sustained clinical pain. Top row shows the results of a meta-analysis of functional neuroimaging publications that frequently use the term ‘pain’ in the full text (Neurosynth-generated ‘reverse inference map’ using the term ‘pain’). The mask highlights the brain regions that are frequently often observed in ‘pain’ studies as compared to studies that do not frequently mention the term ‘pain’. The second row shows the overlap between this Neurosynth mask and the brain areas showing a significant BOLD response when transient painful stimuli are delivered to the right foot of healthy volunteers [data from Mouraux et al. (2011)]. Note the strong overlap, in yellow. The third row shows the BOLD response elicited by a transient painful stimulus in a group of patients with low back pain, generally similar to the BOLD response observed in healthy participants [data from Baliki et al. (2006)]. The bottom row shows, in the same patients, the regions where the BOLD signal correlates significantly with spontaneous fluctuations of low back pain. Note the lack of overlap between these areas and the Neurosynth-generated mask, indicating that a brain biomarker derived from brain activity triggered by acute painful stimuli is likely to be unable to assess pain in clinical conditions. LBP = low back pain.
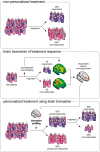
Use of functional neuroimaging to predict treatment response and stratify patients. Non-personalized treatment (top). When a population of patients is exposed to treatment X, some individuals respond to the treatment (e.g. 40%) and others do not (e.g. 60%). Brain biomarker of treatment response (middle). Functional brain imaging before any treatment is delivered to a group of patients to obtain predictive information about whether the patients are responders or non-responders. Personalized treatment using brain biomarker (bottom). The features of brain activity that distinguished responders from non-responders can be looked for in a new population of patients who have not yet been treated, to predict individual patient’s response, and thereby provide the first-line treatment that is most likely to be effective for that individual patient.
Similar articles
-
Recent advances in objectifying pain using neuroimaging techniques.
Archibald J, Warner FM, Ortiz O, Todd M, Jutzeler CR. Archibald J, et al. J Neurophysiol. 2018 Aug 1;120(2):387-390. doi: 10.1152/jn.00171.2018. Epub 2018 May 16. J Neurophysiol. 2018. PMID: 29766766 Free PMC article. Review.
-
Advances and challenges in neuroimaging-based pain biomarkers.
Zhang LB, Chen YX, Li ZJ, Geng XY, Zhao XY, Zhang FR, Bi YZ, Lu XJ, Hu L. Zhang LB, et al. Cell Rep Med. 2024 Oct 15;5(10):101784. doi: 10.1016/j.xcrm.2024.101784. Epub 2024 Oct 8. Cell Rep Med. 2024. PMID: 39383872 Free PMC article. Review.
-
Martucci KT, Mackey SC. Martucci KT, et al. Anesthesiology. 2018 Jun;128(6):1241-1254. doi: 10.1097/ALN.0000000000002137. Anesthesiology. 2018. PMID: 29494401 Free PMC article. Review.
-
Imaging vs quantitative sensory testing to predict chronic pain treatment outcomes.
Davis KD. Davis KD. Pain. 2019 May;160 Suppl 1:S59-S65. doi: 10.1097/j.pain.0000000000001479. Pain. 2019. PMID: 31008851 Review.
-
Kumbhare DA, Elzibak AH, Noseworthy MD. Kumbhare DA, et al. Clin J Pain. 2017 Apr;33(4):281-290. doi: 10.1097/AJP.0000000000000415. Clin J Pain. 2017. PMID: 27518493 Review.
Cited by
-
Bedside Neuromodulation of Persistent Pain and Allodynia with Caloric Vestibular Stimulation.
Ngo TT, Barsdell WN, Law PCF, Arnold CA, Chou MJ, Nunn AK, Brown DJ, Fitzgerald PB, Gibson SJ, Miller SM. Ngo TT, et al. Biomedicines. 2024 Oct 16;12(10):2365. doi: 10.3390/biomedicines12102365. Biomedicines. 2024. PMID: 39457677 Free PMC article.
-
A Comprehensive Review of Pain Interference on Postural Control: From Experimental to Chronic Pain.
Viseux FJF, Simoneau M, Billot M. Viseux FJF, et al. Medicina (Kaunas). 2022 Jun 16;58(6):812. doi: 10.3390/medicina58060812. Medicina (Kaunas). 2022. PMID: 35744075 Free PMC article. Review.
-
Electroencephalography-Based Effects of Acute Alcohol Intake on the Pain Matrix.
Dreismickenbecker E, Zinn S, Romero-Richter M, Kohlhaas M, Fricker LR, Petzel-Witt S, Walter C, Kreuzer M, Toennes SW, Anders M. Dreismickenbecker E, et al. Brain Sci. 2023 Nov 30;13(12):1659. doi: 10.3390/brainsci13121659. Brain Sci. 2023. PMID: 38137107 Free PMC article.
-
Multiple Functional Brain Networks Related to Pain Perception Revealed by fMRI.
Damascelli M, Woodward TS, Sanford N, Zahid HB, Lim R, Scott A, Kramer JK. Damascelli M, et al. Neuroinformatics. 2022 Jan;20(1):155-172. doi: 10.1007/s12021-021-09527-6. Epub 2021 Jun 8. Neuroinformatics. 2022. PMID: 34101115 Free PMC article.
-
SOMAScience: A Novel Platform for Multidimensional, Longitudinal Pain Assessment.
Gunsilius CZ, Heffner J, Bruinsma S, Corinha M, Cortinez M, Dalton H, Duong E, Lu J, Omar A, Owen LLW, Roarr BN, Tang K, Petzschner FH. Gunsilius CZ, et al. JMIR Mhealth Uhealth. 2024 Jan 12;12:e47177. doi: 10.2196/47177. JMIR Mhealth Uhealth. 2024. PMID: 38214952 Free PMC article.
References
-
- Albe-Fessard D, Berkley KJ, Kruger L, Ralston HJ, 3rd, Willis WD Jr. Diencephalic mechanisms of pain sensation. Brain Res 1985; 356: 217–96. - PubMed
-
- Apkarian AV, Bushnell MC, Treede RD, Zubieta JK. Human brain mechanisms of pain perception and regulation in health and disease. Eur J Pain 2005; 9: 463–84. - PubMed
-
- Aru J, Bachmann T, Singer W, Melloni L. Distilling the neural correlates of consciousness. Neurosci Biobehav Rev 2012; 36: 737–46. - PubMed
-
- Baier B, Eulenburg PZ, Geber C, Rohde F, Rolke R, Maihöfner C et al. . Insula and sensory insular cortex and somatosensory control in patients with insular stroke. Eur J Pain 2014; 18: 1385–93. - PubMed
Publication types
MeSH terms
Substances
LinkOut - more resources
Full Text Sources
Other Literature Sources
Medical