Antibiotic Resistance Mechanisms in Bacteria: Relationships Between Resistance Determinants of Antibiotic Producers, Environmental Bacteria, and Clinical Pathogens - PubMed
- ️Mon Jan 01 2018
Review
Antibiotic Resistance Mechanisms in Bacteria: Relationships Between Resistance Determinants of Antibiotic Producers, Environmental Bacteria, and Clinical Pathogens
Elizabeth Peterson et al. Front Microbiol. 2018.
Abstract
Emergence of antibiotic resistant pathogenic bacteria poses a serious public health challenge worldwide. However, antibiotic resistance genes are not confined to the clinic; instead they are widely prevalent in different bacterial populations in the environment. Therefore, to understand development of antibiotic resistance in pathogens, we need to consider important reservoirs of resistance genes, which may include determinants that confer self-resistance in antibiotic producing soil bacteria and genes encoding intrinsic resistance mechanisms present in all or most non-producer environmental bacteria. While the presence of resistance determinants in soil and environmental bacteria does not pose a threat to human health, their mobilization to new hosts and their expression under different contexts, for example their transfer to plasmids and integrons in pathogenic bacteria, can translate into a problem of huge proportions, as discussed in this review. Selective pressure brought about by human activities further results in enrichment of such determinants in bacterial populations. Thus, there is an urgent need to understand distribution of resistance determinants in bacterial populations, elucidate resistance mechanisms, and determine environmental factors that promote their dissemination. This comprehensive review describes the major known self-resistance mechanisms found in producer soil bacteria of the genus Streptomyces and explores the relationships between resistance determinants found in producer soil bacteria, non-producer environmental bacteria, and clinical isolates. Specific examples highlighting potential pathways by which pathogenic clinical isolates might acquire these resistance determinants from soil and environmental bacteria are also discussed. Overall, this article provides a conceptual framework for understanding the complexity of the problem of emergence of antibiotic resistance in the clinic. Availability of such knowledge will allow researchers to build models for dissemination of resistance genes and for developing interventions to prevent recruitment of additional or novel genes into pathogens.
Keywords: Streptomyces; antibiotic resistance; clinical pathogens; environmental bacteria; horizontal gene transfer; producer bacteria; resistance gene dissemination; self-resistance mechanisms.
Figures
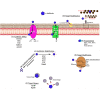
Schematic representation of different antibiotic resistance mechanisms in bacteria, shown with examples. (A) Antibiotic modification involves the addition of acetyl, phosphate, or adenyl groups to aminoglycosides by N-acetyl transferases (AAC), O-phosphotransferases (APH), and O-adenyltransferases (ANT). Other examples include chloramphenicol acetyl transferases (CAT) and bleomycin N-acetyltransferases (BlmB). (B) Antibiotic degradation is observed with β-lactamases, which hydrolyze the antibiotic. (C) Antibiotic efflux pumps remove the antibiotic from the cell using energy from ATP hydrolysis in ABC pumps like DrrAB, OtrC, TlrC, and MlbYZ, or proton gradients in MFS, MATE, SMR, and RND family pumps. (D) Target modification includes various target alterations, such as 23S rRNA or 16S rRNA methylation, alterations in the peptidoglycan precursors (for example, in the case of glycopeptides), or synthesis of alternate low-affinity targets (PBPs) that reduce or completely block antibiotic (penicillins) from associating with the target. (E) Antibiotic sequestration involves proteins that can associate with the antibiotic and block them from reaching their targets. (F) Target bypass involves generation of additional antibiotic targets or subunits that are not susceptible to binding of the antibiotic. Meth, methylation.
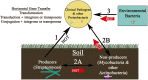
Schematic showing reservoirs of antibiotic resistance genes found in nature and various pathways for their movement to the clinic. Transfer of resistance genes to clinical isolates could occur by a variety of routes (shown by arrows), each using horizontal gene transfer mechanisms potentially involving plasmids, integrons, or transposons. While direct transfer of resistance determinants from producers in the soil to clinical strains is possible (Route 1), a more likely route may first involve movement from the producer soil bacteria to non-producer soil bacteria (for example Mycobacterium species) (Pang et al., 1994) (Route 2A), followed by transfer to clinical pathogens through several carriers (Route 2B). Another, possibly more important route, could involve direct transfer from environmental bacteria (found in bodies of water, aquaculture, livestock animals, wildlife, and plants) to clinical isolates (Route 3). Routes 2 and 3 are shown as thick red arrows, implying greater probability of these pathways for dissemination of resistance genes to clinical strains.
Similar articles
-
Hossain AKMZ, Chowdhury AMMA. Hossain AKMZ, et al. J Basic Microbiol. 2024 Oct;64(10):e2400259. doi: 10.1002/jobm.202400259. Epub 2024 Aug 7. J Basic Microbiol. 2024. PMID: 39113256 Review.
-
Comparison of Antibiotic Resistance Mechanisms in Antibiotic-Producing and Pathogenic Bacteria.
Ogawara H. Ogawara H. Molecules. 2019 Sep 21;24(19):3430. doi: 10.3390/molecules24193430. Molecules. 2019. PMID: 31546630 Free PMC article. Review.
-
Fabbretti A, Çapuni R, Giuliodori AM, Cimarelli L, Miano A, Napolioni V, La Teana A, Spurio R. Fabbretti A, et al. mSphere. 2019 Sep 25;4(5):e00554-19. doi: 10.1128/mSphere.00554-19. mSphere. 2019. PMID: 31554724 Free PMC article.
-
The food safety perspective of antibiotic resistance.
McDermott PF, Zhao S, Wagner DD, Simjee S, Walker RD, White DG. McDermott PF, et al. Anim Biotechnol. 2002 May;13(1):71-84. doi: 10.1081/ABIO-120005771. Anim Biotechnol. 2002. PMID: 12212946 Review.
-
The antibiotic resistance "mobilome": searching for the link between environment and clinic.
Perry JA, Wright GD. Perry JA, et al. Front Microbiol. 2013 May 30;4:138. doi: 10.3389/fmicb.2013.00138. eCollection 2013. Front Microbiol. 2013. PMID: 23755047 Free PMC article.
Cited by
-
Abera D, Alemu A, Mihret A, Negash AA, Abegaz WE, Cadwell K. Abera D, et al. PLoS One. 2023 Nov 27;18(11):e0293528. doi: 10.1371/journal.pone.0293528. eCollection 2023. PLoS One. 2023. PMID: 38011148 Free PMC article.
-
Innovative Phospholipid Carriers: A Viable Strategy to Counteract Antimicrobial Resistance.
Nicolosi D, Petronio Petronio G, Russo S, Di Naro M, Cutuli MA, Russo C, Di Marco R. Nicolosi D, et al. Int J Mol Sci. 2023 Nov 3;24(21):15934. doi: 10.3390/ijms242115934. Int J Mol Sci. 2023. PMID: 37958915 Free PMC article. Review.
-
Gavara L, Verdirosa F, Legru A, Mercuri PS, Nauton L, Sevaille L, Feller G, Berthomieu D, Sannio F, Marcoccia F, Tanfoni S, De Luca F, Gresh N, Galleni M, Docquier JD, Hernandez JF. Gavara L, et al. Biomolecules. 2020 Jul 23;10(8):1094. doi: 10.3390/biom10081094. Biomolecules. 2020. PMID: 32717907 Free PMC article.
-
Berdejo D, Merino N, Pagán E, García-Gonzalo D, Pagán R. Berdejo D, et al. Microorganisms. 2020 Jun 22;8(6):937. doi: 10.3390/microorganisms8060937. Microorganisms. 2020. PMID: 32580471 Free PMC article.
-
Chemical genetic approaches for the discovery of bacterial cell wall inhibitors.
Gupta R, Singh M, Pathania R. Gupta R, et al. RSC Med Chem. 2023 Aug 30;14(11):2125-2154. doi: 10.1039/d3md00143a. eCollection 2023 Nov 15. RSC Med Chem. 2023. PMID: 37974958 Free PMC article. Review.
References
-
- Almutairi M. M., Park S. R., Rose S., Hansen D. A., Vazquez-Laslop N., Douthwaite S., et al. (2015). Resistance to ketolide antibiotics by coordinated expression of rRNA methyltransferases in a bacterial producer of natural ketolides. Proc. Natl. Acad. Sci. U.S.A. 112 12956–12961. 10.1073/pnas.1512090112 - DOI - PMC - PubMed
Publication types
LinkOut - more resources
Full Text Sources
Other Literature Sources