Cell-Type-Specific Profiling of Alternative Translation Identifies Regulated Protein Isoform Variation in the Mouse Brain - PubMed
- ️Tue Jan 01 2019
. 2019 Jan 15;26(3):594-607.e7.
doi: 10.1016/j.celrep.2018.12.077.
Allison M Lake 1 , Wei Yang 2 , Chengran Yang 1 , Hendrik Wesseling 3 , Amanda Guise 3 , Ceren Uncu 3 , Jasbir S Dalal 3 , Andrew W Kraft 4 , Jin-Moo Lee 4 , Mark S Sands 5 , Judith A Steen 3 , Joseph D Dougherty 6
Affiliations
- PMID: 30650354
- PMCID: PMC6392083
- DOI: 10.1016/j.celrep.2018.12.077
Cell-Type-Specific Profiling of Alternative Translation Identifies Regulated Protein Isoform Variation in the Mouse Brain
Darshan Sapkota et al. Cell Rep. 2019.
Abstract
Alternative translation initiation and stop codon readthrough in a few well-studied cases have been shown to allow the same transcript to generate multiple protein variants. Because the brain shows a particularly abundant use of alternative splicing, we sought to study alternative translation in CNS cells. We show that alternative translation is widespread and regulated across brain transcripts. In neural cultures, we identify alternative initiation on hundreds of transcripts, confirm several N-terminal protein variants, and show the modulation of the phenomenon by KCl stimulation. We also detect readthrough in cultures and show differential levels of normal and readthrough versions of AQP4 in gliotic diseases. Finally, we couple translating ribosome affinity purification to ribosome footprinting (TRAP-RF) for cell-type-specific analysis of neuronal and astrocytic translational readthrough in the mouse brain. We demonstrate that this unappreciated mechanism generates numerous and diverse protein isoforms in a cell-type-specific manner in the brain.
Keywords: Aqp4; brain; cell-type specific; gliosis; initiation; neuronal activity; protein variants; readthrough; translation.
Copyright © 2018 The Author(s). Published by Elsevier Inc. All rights reserved.
Conflict of interest statement
DECLARATION OF INTERESTS
J.D.D. has received royalties related to TRAP in the past.
Figures
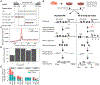
(A) uTISs, aTISs, and dTISs and the corresponding protein products are depicted. uTISs and dTISs give rise to N-terminal variants of the protein when in frame, but code for completely new polypeptide sequences when out of frame. Stop codon readthrough that generates a C-terminal extension is also depicted. In this study, Figures 1, 2, and 3 concern TISs, and Figures 4, 5, and 6 concern readthrough. (B) Experimental workflow for the in vitro study. Mixed neurons and glia from post-natal day (P)0 mice were cultured for 7 days in vitro, exposed to KCl or no KCl, and subjected to RF and TIS-mapping RF as shown. (C) Average ribosomal density across transcripts shows a run-off of elongating ribosomes from the proximal 300-nt region of the coding sequence in the HHT-treated sample as compared to the no-HHT sample. Plot from the KCl-untreated cultures is shown; KCl-treated cultures gave the same density distributions. (D) The number of high-fidelity TISs in the 426 most robustly expressed transcripts. Most of the transcripts showed >1 TIS. Only the top-five TISs were called. (E) Codon composition and frame status of TISs across transcripts. TIS codons are shown as ATG or a cognate thereof (NTG). Numbers inside the bars indicate the percentages of in-frame TISs. RF, ribosome footprinting; HHT, homoharringtonine; TIS, translation initiation site; u/a/dTIS, upstream/annotated/downstream TIS. Also see Figures S1 and S2 and Table S1.
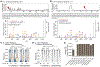
(A and B) Peptides resulting from an in-frame uTIS (Rtn1) (A) and an in-frame dTIS (Uchl1) (B). Upper: the percentage of ribosomes at different TISs (red arrowheads correspond to alternative TISs whose novel products are detected; zeros correspond to aTISs). Middle: the peptide sequences with the amino acids corresponding to alternative TISs and aTISs in red and green, respectively. Bottom: the tandem mass spectra and mass-to-charge ratios of b and y product ions confirming peptide sequences. Alternative TISs for Rtn1 and Uchl1 lie at the −63rd and +15th nt relative to the aTIS, respectively. (C and D) Conservation of uTISs with respect to 5′ UTR (C) and of dTISs with respect to CDS (D). Violin plots show the average of the PhastCons scores for the 3 nt of the ATG, CTG, GTG, or TTG present at the uTISs or dTISs or elsewhere in the 5′ UTR or CDS. Wilcoxon test was used to assess the statistical significance.(E) Conservation of the uTISs and dTISs of mass spectrometry-confirmed peptide products. Plot shows the average of the PhastCons scores of the 3 nucleotides. Also see Tables S1 and S2.
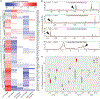
(A) Heatmap shows transcripts with significant changes (false discovery rate ≤0.05) in the usage of aTISs, uTISs, or dTISs in response to KCl depolarization of neuron-glia culture. Usage of a TIS was computed as the P site counts at that TIS divided by the total P site counts across all TISs for a given transcript. For transcripts with multiple uTISs and dTISs, combined changes over all uTISs and dTISs is shown. Chi-square was used to test for the difference of P site counts across TISs. (B) Representative tracts are shown to highlight the abundance of ribosomes at TISs and the use of different TISs in response to KCl depolarization. Numbers on the x axis indicate nucleotide positions, with zero being the aTIS, and numbers on the y axis indicate the percentages of ribosomes. Arrowheads point to the uTISs and dTISs. (C) Boxplot quantifies the KCl-mediated shifts in TIS use of the transcripts shown in (B). n = 3, paired t test. Error bar, SEM. Also see Table S1.
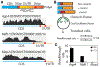
(A) A schematic of footprint mapping and genome browser tracts with examples of readthrough are shown. Aqp4 and Mdh1 display footprints mapping to the readthrough region, whereas Map2 does not. (B) Dual-luciferase assay assessing the permissiveness of sequences for readthrough. A cassette spanning the distal CDS and the readthrough region is cloned between the Renilla luciferase (RL) and firefly luciferase (FL) such that the latter is expressed in transfected cells only if ribosomes read past the cassette. The stop codon is mutated to a sense codon in the positive control, whereas an extra stop codon is added in the negative control. (C) Dual-luciferase assay shows that Aqp4 and Mdh1 undergo readthrough at the rates of 12.5% and 5.5%, respectively, and that Map2 does not undergo readthrough. Readthrough rate is calculated as shown in (B) (n = 3; error bar, SEM). CDS, coding sequence; RA, Renilla activity; FA, firefly activity; Ct, control. Also see Table S3 and Figure S3.
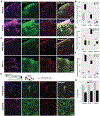
(A, C, and E) Immunostaining for AQP4, AQP4X, and GFAP in brain sections from mice with middle cerebral artery occlusion (A), infantile Batten disease (C), and injury-induced gliosis (E). Normal and gliotic hemispheres of the same sections are imaged in (A) and (E), and age-matched wild-type littermate controls are used in (C). (B, D, and F) Fluorescence intensities in (A), (C), and (E) quantified in (B), (D), and (F), respectively, show that AQP4 is significantly more upregulated than AQP4X by gliosis. Regions of interest were drawn to cover the gliotic area and the contralateral normal area in (A), whereas whole images were considered in (B) and (C). 3 mice, 6 sections/mouse, paired t test; error bar, SEM. (G) Barplot compiles the relative upregulations of AQP4 and AQP4X from (B), (D), and (F). Scale bars, 20 μm. Also see Figures S4 and S5.
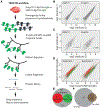
(A) TRAP-RF workflow. mRNAs from transgenic brains expressing GFP-tagged ribosomes in neurons (Snap25::Rpl10a-Egfp) or astrocytes (Aldh1l1::Rpl10a-Egfp) are affinity purified with anti-GFP-conjugated magnetic beads, and subjected to on-bead digestion with RNase and the subsequent RF protocol as shown. (B and C) Log2 (reads per kilobase million [RPKM]) of ribosome footprints mapping to the coding sequence (B) and proximal 3′ UTR (C) was reproducible between the replicate samples for both Snap25::Rpl10a-Egfp and Aldh1l1::Rpl10a-Egfp. (D) Comparison of TRAP-RF samples between neurons and astrocytes shows the expected enrichment of neuronal (green) and astrocytic (red) transcripts identified in previous experiments (Dougherty et al., 2012). (E) Of the 50 transcripts detected undergoing ≥1% readthrough in the brain, 21 are neuronal, 19 are astrocytic, and the remaining 10 are non-cell-type specific. (F) Of the 18 transcripts with ≥1% readthrough in neuron-glia culture, 13 do so in vivo as well. IP, immunoprecipitation. Also see Table S4 and Figures S3 and S6.
Similar articles
-
Van Damme P, Gawron D, Van Criekinge W, Menschaert G. Van Damme P, et al. Mol Cell Proteomics. 2014 May;13(5):1245-61. doi: 10.1074/mcp.M113.036442. Epub 2014 Mar 12. Mol Cell Proteomics. 2014. PMID: 24623590 Free PMC article.
-
Di Bartolomei G, Scheiffele P. Di Bartolomei G, et al. Methods Mol Biol. 2022;2537:37-49. doi: 10.1007/978-1-0716-2521-7_3. Methods Mol Biol. 2022. PMID: 35895257
-
Bowling A, Eastman A, Merlo C, Lin G, West N, Patel S, Cutting G, Sharma N. Bowling A, et al. J Pers Med. 2022 Sep 1;12(9):1448. doi: 10.3390/jpm12091448. J Pers Med. 2022. PMID: 36143233 Free PMC article.
-
Deciphering the molecular mechanism of stop codon readthrough.
Palma M, Lejeune F. Palma M, et al. Biol Rev Camb Philos Soc. 2021 Feb;96(1):310-329. doi: 10.1111/brv.12657. Epub 2020 Oct 22. Biol Rev Camb Philos Soc. 2021. PMID: 33089614 Review.
-
Mammalian proteome expansion by stop codon readthrough.
Manjunath LE, Singh A, Som S, Eswarappa SM. Manjunath LE, et al. Wiley Interdiscip Rev RNA. 2023 Mar;14(2):e1739. doi: 10.1002/wrna.1739. Epub 2022 May 15. Wiley Interdiscip Rev RNA. 2023. PMID: 35570338 Review.
Cited by
-
Karki P, Carney TD, Maracci C, Yatsenko AS, Shcherbata HR, Rodnina MV. Karki P, et al. Nucleic Acids Res. 2022 Jun 24;50(11):6001-6019. doi: 10.1093/nar/gkab1189. Nucleic Acids Res. 2022. PMID: 34897510 Free PMC article.
-
Ribosome profiling: a powerful tool in oncological research.
Su D, Ding C, Qiu J, Yang G, Wang R, Liu Y, Tao J, Luo W, Weng G, Zhang T. Su D, et al. Biomark Res. 2024 Jan 25;12(1):11. doi: 10.1186/s40364-024-00562-4. Biomark Res. 2024. PMID: 38273337 Free PMC article. Review.
-
Loughran G, Li X, O'Loughlin S, Atkins JF, Baranov PV. Loughran G, et al. Nucleic Acids Res. 2023 Jan 11;51(1):304-314. doi: 10.1093/nar/gkac1180. Nucleic Acids Res. 2023. PMID: 36533511 Free PMC article.
-
Rules are made to be broken: a "simple" model organism reveals the complexity of gene regulation.
Higdon AL, Brar GA. Higdon AL, et al. Curr Genet. 2021 Feb;67(1):49-56. doi: 10.1007/s00294-020-01121-8. Epub 2020 Nov 1. Curr Genet. 2021. PMID: 33130938 Free PMC article. Review.
-
Insights Into Translatomics in the Nervous System.
Zhang S, Chen Y, Wang Y, Zhang P, Chen G, Zhou Y. Zhang S, et al. Front Genet. 2020 Dec 21;11:599548. doi: 10.3389/fgene.2020.599548. eCollection 2020. Front Genet. 2020. PMID: 33408739 Free PMC article. Review.
References
-
- Bading H, Ginty DD, and Greenberg ME (1993). Regulation of gene expression in hippocampal neurons by distinct calcium signaling pathways. Science 260, 181–186. - PubMed
-
- Bible E, Gupta P, Hofmann SL, and Cooper JD (2004). Regional and cellular neuropathology in the palmitoyl protein thioesterase-1 null mutant mouse model of infantile neuronal ceroid lipofuscinosis. Neurobiol. Dis 16, 346–359. - PubMed
-
- Bilguvar K, Tyagi NK, Ozkara C, Tuysuz B, Bakircioglu M, Choi M, Delil S, Caglayan AO, Baranoski JF, Erturk O, et al. (2013). Recessive loss of function of the neuronal ubiquitin hydrolase UCHL1 leads to early-onset progressive neurodegeneration. Proc. Natl. Acad. Sci. USA 110, 3489–3494. - PMC - PubMed
Publication types
MeSH terms
Substances
Grants and funding
LinkOut - more resources
Full Text Sources
Other Literature Sources
Molecular Biology Databases
Miscellaneous