Exploratory Cortex Metabolic Profiling Revealed the Sedative Effect of Amber in Pentylenetetrazole-Induced Epilepsy-Like Mice - PubMed
- ️Tue Jan 01 2019
Exploratory Cortex Metabolic Profiling Revealed the Sedative Effect of Amber in Pentylenetetrazole-Induced Epilepsy-Like Mice
Zhenhua Zhu et al. Molecules. 2019.
Abstract
Epilepsy is a common clinical syndrome characterized by sudden and recurrent attacks and temporary central nervous system dysfunction caused by excessive discharge of neurons in the brain. Amber, a fossilized organic substance formed by the resins of conifers and leguminous plants, was prescribed to tranquilize the mind in China. In this paper, the antiepileptic effect of amber was evaluated by a pentylenetetrazole (PTZ)-induced epileptic model. An untargeted metabolomics approach was applied to investigate metabolic changes in the epileptic model, which was based on HILIC-UHPLC-MS/MS multivariate statistical analysis and metabolism network analysis. The outcome of this study suggested that 35 endogenous metabolites showed marked perturbations. Moreover, four metabolism pathways were mainly involved in epilepsy. After treatment by amber, the endogenous metabolites had a marked tendency to revert back to the situation of the control group which was consistent with phenobarbital. This study characterized the pentylenetetrazole-induced epileptic model and provided new evidence for the sedative effect of amber.
Keywords: LC/MS; amber; epilepsy; glycerophospholipid metabolism; metabolomics.
Conflict of interest statement
The authors declare no conflict of interest.
Figures
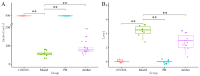
The effect of amber on behavior in the seizure model induced by pentylenetetrazole. (A) The incubation time of each group. Control group and PB group had no incubation time, and used 900 s for analysis. Under the intervention of amber, the incubation period was significantly prolonged compared to the model group. (B) Under the intervention of amber, the level of seizures was significantly reduced compared to the model group. ** p < 0.01 for extremely significant difference.
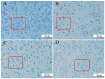
Amber rescues CA1 pyramidal neurons from seizure-induced damage as revealed by Nissl staining. (A) Control group; (B) Model group (PTZ); (C) PB group (PTZ + Phenobarbital); (D) Amber group (PTZ + amber). Photomicrographs show sample CA1 subfields (magnification, ×400) in the coronal plane for each treatment group. A damaged cell body is indicated by red frame. These signs of neural damage were reduced by amber pre-treatment.
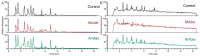
Base peak chromatograms (BPCs) of cortex samples of control group, model group, and amber group under ESI+ (A) and ESI− (B) MS conditions.
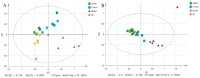
PCA scores scatter plot for control group, model group and amber group. (A) positive mode, R2X (cum) = 0.641, Q2 (cum) = 0.461; (B) negative mode, R2X (cum) = 0.836, Q2 (cum) = 0.449.
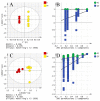
OPLS-DA score plot and validation plot of the OPLS-DA model of control group and model group. OPLS-DA score plot for the first two components showed the separation between the control group and model group. The fitness (R2Y) and prediction power (Q2Y) of this two-component model were 0.979 and 0.918, respectively ((A) ESI+; (C) ESI−). Validation plot of the OPLS-DA model of control group and model group were obtained from 200 permutation tests. The intercepts of R2 were lower than the original point to the right, whereas those of Q2 were negative, indicating no signs of overfit ((B) ESI+; (D) ESI−).
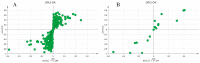
Loading S-plots generated by OPLS-DA analysis in positive mode (A) and negative mode (B). The x-axis is a measure of the relative abundance of ions, and the y-axis is a measure of the correlation of each ion to the model.
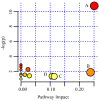
Summary of pathway analysis with MetPA. (A) glycerophospholipid metabolism; (B) nicotinate and nicotinamide metabolism; (C) alanine, aspartate and glutamate metabolism; (D) pyruvate metabolism.
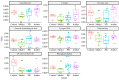
Boxplot of the metabolites associated with the four pathways in Figure 7.
Similar articles
-
Wolahan SM, Hirt D, Glenn TC. Wolahan SM, et al. In: Kobeissy FH, editor. Brain Neurotrauma: Molecular, Neuropsychological, and Rehabilitation Aspects. Boca Raton (FL): CRC Press/Taylor & Francis; 2015. Chapter 25. In: Kobeissy FH, editor. Brain Neurotrauma: Molecular, Neuropsychological, and Rehabilitation Aspects. Boca Raton (FL): CRC Press/Taylor & Francis; 2015. Chapter 25. PMID: 26269925 Free Books & Documents. Review.
-
Wei C, Li Y, Yao H, Liu H, Zhang X, Guo R. Wei C, et al. Mol Biosyst. 2012 Aug;8(8):2197-204. doi: 10.1039/c2mb25105a. Epub 2012 Jun 15. Mol Biosyst. 2012. PMID: 22706165
-
Myelin damage of hippocampus and cerebral cortex in rat pentylenetetrazol model.
You Y, Bai H, Wang C, Chen LW, Liu B, Zhang H, Gao GD. You Y, et al. Brain Res. 2011 Mar 24;1381:208-16. doi: 10.1016/j.brainres.2011.01.011. Epub 2011 Jan 20. Brain Res. 2011. PMID: 21256118
-
Bruno C, Patin F, Bocca C, Nadal-Desbarats L, Bonnier F, Reynier P, Emond P, Vourc'h P, Joseph-Delafont K, Corcia P, Andres CR, Blasco H. Bruno C, et al. J Pharm Biomed Anal. 2018 Jan 30;148:273-279. doi: 10.1016/j.jpba.2017.10.013. Epub 2017 Oct 18. J Pharm Biomed Anal. 2018. PMID: 29059617
-
Löscher W. Löscher W. Neurochem Res. 2017 Jul;42(7):1926-1938. doi: 10.1007/s11064-016-2025-7. Epub 2016 Aug 8. Neurochem Res. 2017. PMID: 27502939 Review.
Cited by
-
Lalwani AM, Yilmaz A, Bisgin H, Ugur Z, Akyol S, Graham SF. Lalwani AM, et al. Metabolites. 2020 Jun 23;10(6):261. doi: 10.3390/metabo10060261. Metabolites. 2020. PMID: 32585915 Free PMC article.
-
Wei C, Zhu Z, Zheng JN, Lu Y, Cao C, Qu S, Liu M, Meng XE, Lou Q, Wang Q, Duan JA, Shang EX, Han Z, Zhu Y. Wei C, et al. Front Pharmacol. 2022 Jun 15;13:867477. doi: 10.3389/fphar.2022.867477. eCollection 2022. Front Pharmacol. 2022. PMID: 35784758 Free PMC article.
-
Shen J, Li YZ, Yao S, Zhu ZW, Wang X, Sun HH, Ji WF. Shen J, et al. Front Pharmacol. 2022 Jul 18;13:952696. doi: 10.3389/fphar.2022.952696. eCollection 2022. Front Pharmacol. 2022. PMID: 35924045 Free PMC article.
-
Characterization and antimicrobial study of Trinakantamani (Amber) Pishti.
Joshi N, Ahuja MR, Rastogi GK, Dash MK. Joshi N, et al. Ayu. 2020 Oct-Dec;41(4):225-234. doi: 10.4103/ayu.AYU_155_19. Epub 2022 Jun 3. Ayu. 2020. PMID: 35813362 Free PMC article.
-
Lu H, Wang Q, Jiang X, Zhao Y, He M, Wei M. Lu H, et al. Molecules. 2023 Mar 20;28(6):2805. doi: 10.3390/molecules28062805. Molecules. 2023. PMID: 36985783 Free PMC article.
References
-
- Tomson T., Battino D., Bonizzoni E., Craig J., Lindhout D., Sabers A., Perucca E., Vajda F. Dose-dependent risk of malformations with antiepileptic drugs: An analysis of data from the EURAP epilepsy and pregnancy registry. Lancet Neurol. 2011;10:609–617. doi: 10.1016/S1474-4422(11)70107-7. - DOI - PubMed
MeSH terms
Substances
LinkOut - more resources
Full Text Sources
Medical