Comparative Loss-of-Function Screens Reveal ABCE1 as an Essential Cellular Host Factor for Efficient Translation of Paramyxoviridae and Pneumoviridae - PubMed
- ️Tue Jan 01 2019
Comparative Study
. 2019 May 14;10(3):e00826-19.
doi: 10.1128/mBio.00826-19.
Kristin Pfeffermann # 2 , So Young Kim 3 4 , Bevan Sawatsky 2 , James Pearson 3 4 , Mikhail Kovtun 3 , David L Corcoran 3 , Yvonne Krebs 2 , Kristmundur Sigmundsson 5 , Sharon F Jamison 3 , Zhen Zhen Joanna Yeo 1 , Linda J Rennick 6 , Lin-Fa Wang 1 , Pierre J Talbot 7 , W Paul Duprex 6 , Mariano A Garcia-Blanco 8 3 4 9 , Veronika von Messling 8 2 7
Affiliations
- PMID: 31088929
- PMCID: PMC6520455
- DOI: 10.1128/mBio.00826-19
Comparative Study
Comparative Loss-of-Function Screens Reveal ABCE1 as an Essential Cellular Host Factor for Efficient Translation of Paramyxoviridae and Pneumoviridae
Danielle E Anderson et al. mBio. 2019.
Abstract
Paramyxoviruses and pneumoviruses have similar life cycles and share the respiratory tract as a point of entry. In comparative genome-scale siRNA screens with wild-type-derived measles, mumps, and respiratory syncytial viruses in A549 cells, a human lung adenocarcinoma cell line, we identified vesicular transport, RNA processing pathways, and translation as the top pathways required by all three viruses. As the top hit in the translation pathway, ABCE1, a member of the ATP-binding cassette transporters, was chosen for further study. We found that ABCE1 supports replication of all three viruses, confirming its importance for viruses of both families. More detailed characterization revealed that ABCE1 is specifically required for efficient viral but not general cellular protein synthesis, indicating that paramyxoviral and pneumoviral mRNAs exploit specific translation mechanisms. In addition to providing a novel overview of cellular proteins and pathways that impact these important pathogens, this study highlights the role of ABCE1 as a host factor required for efficient paramyxovirus and pneumovirus translation.IMPORTANCE The Paramyxoviridae and Pneumoviridae families include important human and animal pathogens. To identify common host factors, we performed genome-scale siRNA screens with wild-type-derived measles, mumps, and respiratory syncytial viruses in the same cell line. A comparative bioinformatics analysis yielded different members of the coatomer complex I, translation factors ABCE1 and eIF3A, and several RNA binding proteins as cellular proteins with proviral activity for all three viruses. A more detailed characterization of ABCE1 revealed its essential role for viral protein synthesis. Taken together, these data sets provide new insight into the interactions between paramyxoviruses and pneumoviruses and host cell proteins and constitute a starting point for the development of broadly effective antivirals.
Keywords: ABCE1; Paramyxoviridae; Pneumoviridae; RNAi screen; host factor; respiratory syncytial virus.
Copyright © 2019 Anderson et al.
Figures
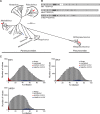
Selection of viruses and RNAi screen performance. (A) A total of 45 N protein sequences were downloaded from GenBank: measles virus (MeV), rinderpest virus (RPV), canine distemper virus (CDV), pestes-des-petits ruminants virus (PPRV), phocine distemper virus (PDV), cetacean morbillivirus virus (CeMV), mumps virus (MuV), human parainfluenza viruses 1 to 4 (hPIV1 to -4), porcine rubulavirus (PorPV), Mapuera virus (MprPV), parainfluenza virus 5 (PIV5), Menangle virus (MenPV), Tioman virus (TioPV), Newcastle disease virus (NDV) avian paramyxoviruses 2 to 9 (APMV 2 to -9), Sendai virus (SeV), bovine parainfluenza virus 3 (bPIV3), Hendra virus (HeV), Nipah virus (NiV), J paramyxovirus (JPV), Beilong paramyxovirus (BeiPV), Salem virus (SalPV), Tupaia paramyxovirus (TupPV), Nariva virus (NarPV), Mossman virus (MosPV), fer-de-lance virus (FdlPV), Atlantic salmon paramyxovirus (AsaPV), bovine respiratory syncytial virus (BRSV), human respiratory syncytial virus (HRSV), avian metapneumovirus (aMPV), and human metapneumovirus (hMPV). Amino acids were aligned using the MUSCLE plugin in Geneious 7.1.6. Alignments were visually inspected and manually curated. The best protein evolution model was determined according to Akaike information criterion (AIC) scores using ProtTest. An LG+G+F maximum likelihood analysis was run in PHYML using average likelihood ratio test (aLRT) statistics for branch support. MeV, MuV, and HRSV are boldfaced and highlighted with a thick red arrow to indicate that the viruses were screened, and HeV is boldfaced and highlighted with a thin blue arrow to indicate that data from a separate screen (43) were included in candidate host factor selection. (B) Schematic drawing of recombinant viruses. Gray and white boxes represent open reading frames and UTRs, respectively. The genes are indicated by their respective abbreviations. The inserted EGFP gene is shaded in dark gray, and the number in parentheses indicates the position of the gene in the viral genome. (C) Histogram of percent infection distribution for MeV, MuV, and HRSV. Distribution of percent infection values for each of the individual virus screens. For reference, distribution of positive (red), negative (blue), and uninfected (gray) controls is shown for each screen.
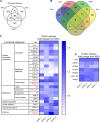
Identification and validation of common proviral hits. (A) Common Paramyxoviridae and Pneumoviridae host factors. Extent of overlap among the genes required for the replication of MeV, MuV, and RSV. The number of genes inhibiting the replication of the viruses in both A/B and C/D siRNA sets (robust Z score < 0.8) is tabulated in the Venn diagram. (B) Extent of overlap among the genes required for the replication of MeV, MuV, RSV, and HeV. The number of genes inhibiting the replication of the viruses is tabulated in the Venn diagram. Multiple siRNAs were used to target each of the six top-ranking genes identified by comparative analysis of the three primary screens and the previously published HeV screen (43). (C) MuV validation screen of 24 common hits identified by both Z score and KS analysis. Forty-eight hours after siRNA transfection, cells were infected with MuV at a multiplicity of infection of 1.0 and incubated for 48 h. The percentage of infected cells was calculated, and the mean percent infection was set at 1 for the NSC. The heat map shows the fold change compared to the NSC, and each square represents the average from three experiments for each individual siRNA. Functional categorization of genes is shown beside the heat map. The gene hits shown in red were then selected for MeV validation screening. (D) MeV validation screen of top proviral hits also identified in the HeV screen. Forty-eight hours after siRNA transfection, cells were infected with MeV at a multiplicity of infection of 0.05 and incubated for 48 h. The percentage of infected cells was calculated, and the mean percent infection was set at 1 for the NSC. The heat map shows the fold change compared to the NSC, and each square represents the average from five experiments for each individual siRNA.
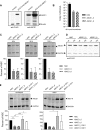
ABCE1 protein expression levels and knockdown efficiency. (A) Confirmation of rabbit anti-ABCE1 peptide antiserum specificity. A549-hSLAM cells were transfected with an expression plasmid coding for human ABCE1 containing a FLAG tag (DYKDDDDK) at the N terminus or left untransfected. After 24 h, cells were lysed in RIPA buffer and clarified lysates were separated by SDS-PAGE and transferred to PVDF membranes. Western blot analyses were performed with either the rabbit anti-ABCE1 serum (right panel) or a monoclonal mouse anti-FLAG antibody (left panel); secondary antibodies against either rabbit or mouse, respectively; and a mouse monoclonal antibody against β-actin directly conjugated to HRP as an internal control. (B) Reduction of ABCE1 mRNA levels following ABCE1_5 or ABCE1_6 siRNA transfection. ABCE1 mRNA copy numbers were quantified by real-time RT-PCR 48 h after siRNA transfection. ABCE1 mRNA copy numbers from three independent replicates were quantified. Error bars represent the standard deviation. (C) Continuous ABCE1 knockdown following ABCE1_5 or ABCE1_6 siRNA transfection. Reduction in cellular ABCE1 protein levels 48 h (left panel), 96 h (center panel), and 120 h (right panel) after siRNA transfection. ABCE1 bands from three independent replicates were quantified and normalized relative to an internal GAPDH control for each blot and are shown as percent reduction of ABCE1 expression relative to the NSC for each time point. Error bars represent the standard deviation. (D) Specificity of ABCE1_5 or ABCE1_6 siRNAs. A549-hSLAM cells were transfected with the FLAG-tagged ABCE1 expression plasmid or a derivative carrying 7 to 10 noncoding mutations in the binding sites of ABCE1_5 and ABCE1_6 siRNAs. After 48 h, the cells were transfected with the respective siRNAs, and ABCE1 protein levels were visualized by Western blotting using a FLAG-specific antibody 48 h later. (E) Validation of siRNA target specificity. Cells were transfected with the respective ABCE1 siRNAs or their corresponding C911 control variants. After 48 h, cells were infected with MeV at an MOI of 0.01. ABCE1 levels in uninfected cells are shown in the left panel, and MeV N levels in MeV-infected cells are shown in the right panel. Protein levels from three independent replicates were quantified, and the NSC value was set to 100%. Error bars represent the standard deviation. *, P < 0.05; **, P < 0.01; ***, P < 0.001.
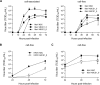
Validation of ABCE1 knockdown effect on MeV, MuV, and RSV replication. Reduction in virus replication as a result of ABCE1 knockdown. (A) A549-hSLAM cells were transfected with control NSC, ABCE1_5, or ABCE1_6 siRNAs for 48 h. Cells were then infected with MeV at an MOI of 0.01. Cell lysates (left panel) and culture supernatants (right panel) were harvested at the indicated time points and titrated on A549-hSLAM cells. (B and C) A549-hSLAM cells were transfected with control NSC or ABCE1_6 siRNAs for 48 h. Cells were then infected with either RSV or MuV at an MOI of 0.01. Supernatant titers are shown for RSV (B) and MuV (C). Each data point represents at least three replicates, and error bars indicate the standard deviation.
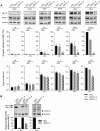
Assessment of ABCE1 knockdown on viral protein and mRNA levels. (A) Kinetics of N protein (top and middle panels) and mRNA (bottom panel) levels over the course of MeV infection at an MOI of 0.01. A549-hSLAM cells were transfected with control NSC, ABCE1_5, or ABCE1_6 siRNAs for 48 h. Cells were then infected, and samples were harvested at the indicated time points. N protein bands from three independent replicates were quantified and normalized relative to an internal GAPDH control for each blot and are shown as percent reduction of N protein expression relative to the NSC for each virus. Copy numbers of N gene mRNA were quantified from the same samples by real-time RT-PCR using a synthetized RNA standard. (B) Reduction of viral N protein expression in siRNA-transfected cells infected with either MeV or MuV (left panel) or RSV (right panel) at either 48 or 72 h postinfection, respectively. MeV, MuV, and RSV N protein bands from three independent replicates were each quantified and normalized relative to an internal GAPDH control for each blot and are shown as the percent reduction of viral N protein expression relative to the NSC for each virus. Error bars represent the standard deviation. ns, P ≥ 0.05; *, P < 0.05; **, P < 0.01; ***, P < 0.001; ****, P < 0.0001.
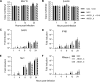
Role of ABCE1 in mRNA transcription and innate immune activation. (A and B) Analysis of de novo mRNA synthesis. A549-hSLAM cells were transfected with control NSC or ABCE1_6 siRNAs for 48 h, infected with MeV at an MOI of 1, and treated with 100 μg/ml CHX or left untreated starting 30 min before infection. Copy numbers of MeV N (A) and β-actin mRNA levels (B) were quantified at different times after infection by real-time RT-PCR using a synthetized RNA standard. (C to F) Evaluation of innate immune response activation. Induction of interferon-related gene expression was assessed by quantifying relative mRNA levels of OAS3 (C), IFN-β (D), Mx1 (E), and RNase L (F). Relative changes in mRNA levels from three independent experiments were quantified by real-time RT-PCR using the ΔΔCT method with GAPDH as standard and are shown relative to the NSC signal at 1 hpi for each cytokine. Error bars indicate the standard deviation, and none of the differences seen reached statistical significance (P > 0.05).
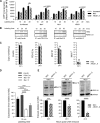
ABCE1 effect on de novo viral protein translation. (A) Quantification of total cellular protein synthesis by incorporation of O-propargyl-puromycin (OPP). At 48 h after siRNA transfection, cells were infected with MeV, MuV, or HRSV for a further 48 h, after which the cells were treated with OPP for the indicated times, and incorporated OPP was coupled to Alexa Fluor 594. The fluorescence signal was quantified by flow cytometry, and bars show the mean fluorescence intensity (MFI). Error bars represent the standard error of the mean. Statistical significance was determined by one-tailed t tests. (B to D) Analysis of protein translation by 35S pulse-labeling and quantification of the corresponding mRNA levels. A549-hSLAM cells were transfected with control NSC or ABCE1_6 siRNAs for 48 h. Cells were then infected with MeV at an MOI of 0.1 and labeled with [35S]Met-Cys 24 h later. (B) Immunoprecipitated cellular proteins β-actin and PDH E1α and MeV M and F proteins from infected cells were visualized by exposure to phosphor screens. Representative phosphor screen images are shown. (C) Copy numbers of the respective mRNAs from three independent experiments corresponding to the 35S pulse-labeling were quantified by real-time RT-PCR using a synthetized RNA standard for each gene. (D) Quantification of 35S pulse-label incorporation. The expression levels in samples shown in panel B from ABCE1_6 siRNA-treated cells relative to NSC-treated cells were calculated for each protein. The average from three independent experiments for the 30-min time point is shown. (E) Analysis of de novo protein synthesis of MeV, MuV, and RSV. A549-hSLAM cells were transfected with control NSC or ABCE1_6 siRNAs for 48 h; infected with MeV, MuV, or HRSV at an MOI of 1; and treated with 100 μg/ml CHX starting 30 min before infection. After 24 h, CHX was removed and samples were harvested after an additional 24 h of incubation. MeV, MuV, and RSV N protein bands were quantified and normalized relative to an internal GAPDH control for each blot and are shown as the percent reduction of viral N protein expression relative to the NSC for each virus. Each graph shows the average from three independent experiments, and error bars represent the standard deviation. Statistical significance in panels C to E is indicated as follows: ns, P ≥ 0.05; ***, P < 0.001; ****, P < 0.0001.
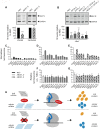
Dependence of ABCE1 proviral effect on viral gene expression during infection. (A) Plasmid-expressed MeV N is not sensitive to ABCE1 knockdown. A549-hSLAM cells were transfected with NSC or ABCE1_6 siRNAs. After 24 h, cells were transfected either with a control empty vector plasmid followed by infection with MeV at an MOI of 0.01 at 24 h posttransfection (“infected”) or with a plasmid expressing MeV N containing an N-terminal FLAG tag and left uninfected 24 posttransfection (“transfected”). The MeV N protein levels were quantified, and NSC was set to 100%. Data are representative of four replicates. Error bars represent standard deviations. Statistical significance is indicated as follows: ns, P ≥ 0.05; ****, P < 0.0001. (B) ABCE1 and eIF3A have similar effects on MeV replication. A549-hSLAM cells were transfected with the respective siRNAs. After 48 h, cells were infected with MeV at an MOI of 0.01. Cell lysates were harvested 48 h after infection. MeV N protein levels were quantified, and NSC was set to 100%. Data are representative of three independent replicates. ns, P ≥ 0.05; *, P < 0.05; **, P < 0.01; ***, P < 0.001. (C to G) Effect of viral 5′ untranslated regions (UTRs) on reporter gene translation. Hep-G2 cells were transfected with either NSC or the ABCE1_5 and ABCE1_6 siRNAs in 96-well plates along with dual-luciferase reporter plasmids. Translation of firefly luciferase (ffLuc) is mediated by upstream cellular or viral UTRs, and the translation of the downstream Renilla luciferase (RenLuc) is driven by the hepatitis C virus (HCV) internal ribosomal entry site (IRES). Luciferase values were read 48 h after transfection. ffLuc signals for each well were normalized to the RenLuc signal. Normalized ffLuc values are shown for cells transfected with NSC (C), ABCE1_5 (D), and ABCE1_6 (E), and the signals from ABCE1_5- and ABCE1_6-transfected cells are shown relative to NSC (F and G, respectively). Transfections in each replicate were performed in triplicate, and all experiments were performed three times. (H) Model for the differential effects of ABCE1 knockdown on viral and cellular protein translation. In cells with normal ABCE1 levels, viral proteins are preferentially translated over cellular proteins, resulting in proportionately higher levels of viral protein expression (top). In cells with reduced ABCE1 levels, viral protein translation is drastically reduced, while translation of cellular proteins is only moderately affected (bottom).
Similar articles
-
Shrestha N, Gall FM, Mathieu C, Hierweger MM, Brügger M, Alves MP, Vesin J, Banfi D, Kalbermatter D, Horvat B, Chambon M, Turcatti G, Fotiadis D, Riedl R, Plattet P. Shrestha N, et al. mBio. 2021 Dec 21;12(6):e0262121. doi: 10.1128/mBio.02621-21. Epub 2021 Nov 2. mBio. 2021. PMID: 34724816 Free PMC article.
-
The essential vertebrate ABCE1 protein interacts with eukaryotic initiation factors.
Chen ZQ, Dong J, Ishimura A, Daar I, Hinnebusch AG, Dean M. Chen ZQ, et al. J Biol Chem. 2006 Mar 17;281(11):7452-7. doi: 10.1074/jbc.M510603200. Epub 2006 Jan 18. J Biol Chem. 2006. PMID: 16421098
-
ABCE1 is essential for S phase progression in human cells.
Toompuu M, Kärblane K, Pata P, Truve E, Sarmiento C. Toompuu M, et al. Cell Cycle. 2016 May 2;15(9):1234-47. doi: 10.1080/15384101.2016.1160972. Epub 2016 Mar 17. Cell Cycle. 2016. PMID: 26985706 Free PMC article.
-
Small hydrophobic (SH) proteins of Pneumoviridae and Paramyxoviridae: small but mighty.
Brynes A, Williams JV. Brynes A, et al. J Virol. 2024 Sep 17;98(9):e0080924. doi: 10.1128/jvi.00809-24. Epub 2024 Aug 23. J Virol. 2024. PMID: 39177356 Review.
-
Phosphorylation of paramyxovirus phosphoprotein and its role in viral gene expression.
Fuentes SM, Sun D, Schmitt AP, He B. Fuentes SM, et al. Future Microbiol. 2010 Jan;5(1):9-13. doi: 10.2217/fmb.09.93. Future Microbiol. 2010. PMID: 20020826 Free PMC article. Review.
Cited by
-
Lu B. Lu B. Front Cell Dev Biol. 2022 Sep 14;10:970654. doi: 10.3389/fcell.2022.970654. eCollection 2022. Front Cell Dev Biol. 2022. PMID: 36187485 Free PMC article. Review.
-
Discovering antiviral restriction factors and pathways using genetic screens.
Jones CE, Tan WS, Grey F, Hughes DJ. Jones CE, et al. J Gen Virol. 2021 May;102(5):001603. doi: 10.1099/jgv.0.001603. J Gen Virol. 2021. PMID: 34020727 Free PMC article. Review.
-
Ammour Y, Susova O, Krasnov G, Nikolaeva E, Varachev V, Schetinina Y, Gavrilova M, Mitrofanov A, Poletaeva A, Bekyashev A, Faizuloev E, Zverev VV, Svitich OA, Nasedkina TV. Ammour Y, et al. Viruses. 2022 Nov 2;14(11):2433. doi: 10.3390/v14112433. Viruses. 2022. PMID: 36366531 Free PMC article.
-
Clinical and biological consequences of respiratory syncytial virus genetic diversity.
Rios Guzman E, Hultquist JF. Rios Guzman E, et al. Ther Adv Infect Dis. 2022 Oct 8;9:20499361221128091. doi: 10.1177/20499361221128091. eCollection 2022 Jan-Dec. Ther Adv Infect Dis. 2022. PMID: 36225856 Free PMC article. Review.
-
Measles Virus-Induced Host Immunity and Mechanisms of Viral Evasion.
Amurri L, Reynard O, Gerlier D, Horvat B, Iampietro M. Amurri L, et al. Viruses. 2022 Nov 26;14(12):2641. doi: 10.3390/v14122641. Viruses. 2022. PMID: 36560645 Free PMC article. Review.
References
-
- Afonso CL, Amarasinghe GK, Bányai K, Bào Y, Basler CF, Bavari S, Bejerman N, Blasdell KR, Briand F-X, Briese T, Bukreyev A, Calisher CH, Chandran K, Chéng J, Clawson AN, Collins PL, Dietzgen RG, Dolnik O, Domier LL, Dürrwald R, Dye JM, Easton AJ, Ebihara H, Farkas SL, Freitas-Astúa J, Formenty P, Fouchier RAM, Fù Y, Ghedin E, Goodin MM, Hewson R, Horie M, Hyndman TH, Jiāng D, Kitajima EW, Kobinger GP, Kondo H, Kurath G, Lamb RA, Lenardon S, Leroy EM, Li C-X, Lin X-D, Liú L, Longdon B, Marton S, Maisner A, Mühlberger E, Netesov SV, Nowotny N, Patterson JL, Payne SL, Paweska JT, Randall RE, Rima BK, Rota P, Rubbenstroth D, Schwemmle M, Shi M, Smither SJ, Stenglein MD, Stone DM, Takada A, Terregino C, Tesh RB, Tian J-H, Tomonaga K, Tordo N, Towner JS, Vasilakis N, Verbeek M, Volchkov VE, Wahl-Jensen V, Walsh JA, Walker PJ, Wang D, Wang L-F, Wetzel T, Whitfield AE, Xiè J, Yuen K-Y, Zhang Y-Z, Kuhn JH. 2016. Taxonomy of the order Mononegavirales: update 2016. Arch Virol 161:2351–2360. doi:10.1007/s00705-016-2880-1. - DOI - PMC - PubMed
-
- Collins PL, Karron RA. 2013. Respiratory syncytial virus and metapneumovirus, p 1086–1123. In Knipe DM, Howley PM, Cohen JI, Griffin DE, Lamb RA, Martin MA, Racaniello VR, Roizman B (ed), Fields virology, 6th ed, vol 1 Lippincott Williams & Wilkins, Philadelphia, PA.
-
- Lamb RA, Parks GD. 2013. Paramyxoviridae, p 957–995. In Knipe DM, Howley PM, Cohen JI, Griffin DE, Lamb RA, Martin MA, Racaniello VR, Roizman B (ed), Fields virology, 6th ed, vol 1 Lippincott Williams & Wilkins, Philadelphia, PA.
Publication types
MeSH terms
Substances
LinkOut - more resources
Full Text Sources
Research Materials