Water oxidation in photosystem II - PubMed
Review
Water oxidation in photosystem II
Wolfgang Lubitz et al. Photosynth Res. 2019 Oct.
Abstract
Biological water oxidation, performed by a single enzyme, photosystem II, is a central research topic not only in understanding the photosynthetic apparatus but also for the development of water splitting catalysts for technological applications. Great progress has been made in this endeavor following the report of a high-resolution X-ray crystallographic structure in 2011 resolving the cofactor site (Umena et al. in Nature 473:55-60, 2011), a tetra-manganese calcium complex. The electronic properties of the protein-bound water oxidizing Mn4OxCa complex are crucial to understand its catalytic activity. These properties include: its redox state(s) which are tuned by the protein matrix, the distribution of the manganese valence and spin states and the complex interactions that exist between the four manganese ions. In this short review we describe how magnetic resonance techniques, particularly EPR, complemented by quantum chemical calculations, have played an important role in understanding the electronic structure of the cofactor. Together with isotope labeling, these techniques have also been instrumental in deciphering the binding of the two substrate water molecules to the cluster. These results are briefly described in the context of the history of biological water oxidation with special emphasis on recent work using time resolved X-ray diffraction with free electron lasers. It is shown that these data are instrumental for developing a model of the biological water oxidation cycle.
Keywords: EPR spectroscopy; Oxygen-evolving complex; Photosystem II; Quantum chemical calculations; Triplet oxygen formation; Water binding.
Conflict of interest statement
The authors declare that they have no conflict of interest.
Figures
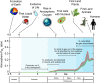
Oxygen build-up in the Earth’s atmosphere on a time scale of billions of years (Ga) and some major events in the development of our planet. The red and green curves denote an upper and lower estimate of the oxygen in the atmosphere. Oxygenic photosynthesis started about ≈ 3.5 Ga ago (Planavsky et al. 2014), the release of O2 in the atmosphere ≈ 2.4 Ga ago (Bekker et al. 2004). The present level of O2 is ≈ 21% (Holland 2006)
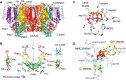
X-ray crystallographic structure of photosystem II (PS II) from T. vulcanus. A View of the dimeric protein (molecular weight of the dimer ≈ 700 kDa); the two monomers are related to each other by a C2 axis. The most important subunits are indicated, CP43, CP47, in which the core antenna pigments are located; D1 and D2, which bind all pigments of the reaction center and all cofactors of the electron transport chain, and the small subunits PsbO, PsbU and PsbV, which stabilize the water splitting unit. The D1 protein (yellow) holds the active branch cofactors and the Mn cluster, the D2 protein (orange) the cofactors of the second pigment branch. B Pigment arrangement in one PS II monomer; the two branches are related by a pseudo-C2 axis (dotted line). Shown are the primary donor P680 (four chlorophylls: PD1, PD2, ChlD1, ChlD2), the two pheophytins (PheoD1, PheoD2), the two plastoquinones (QA, QB), and the non-heme iron Fe. Additional chlorophylls (Chlz) and carotenoids (Car), as well as two redox-active tyrosines (TyrZ, TyrD) are also indicated. Next to the active branch (D1) and close to TyrZ/P680, the Mn4O5Ca cluster is located. C Structure of the water oxidizing Mn4O5Ca cluster in PS II with four Mn ions (Mn1 to Mn4, purple) and one Ca (yellow), bridged by oxygen ligands (red). Three Mn ions (1 to 3) and the Ca form a distorted cube bridged by oxygen ligands, the fourth Mn (Mn4) is dangling. Mn4 and the Ca carry two water molecules each (W1 to W4, orange). The coordination of the metal ions by amino acid ligands is also shown; for further details see (Suga et al. ; Umena et al. 2011). D Water channels leading to the OEC: Three channels have been localized. Note that also the essential chloride ion is shown in this picture (Suga et al. ; Umena et al. 2011). A second Cl− is found farther away from the OEC. It has been pointed out that the channels could be multi-functional
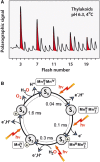
A Release pattern of molecular oxygen (O2) measured polarographically following successive light flashes of spinach thylakoids at 4 °C (Messinger and Renger 2008). Note that O2 release follows a 4-flash pattern (the starting dark stable state is S1). The original experiment was performed by Pierre Joliot as early as 1969 (Joliot et al. 1969). B Water oxidation cycle (Kok cycle) (Kok et al. 1970) detailing the five basic S states (S0 to S4), the light-induced 1e− oxidation steps and the proton release pattern (Dau and Haumann 2008), the uptake of the two substrate waters (Hillier and Wydrzynski 2008) and the Mn oxidation states (Krewald et al. 2015) (vide infra). The reaction times for the single electron oxidation steps are also indicated (Klauss et al. 2012a). Note that here the “S” stands for “state” and not for the electron spin quantum number S
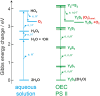
Gibbs energy (in eV) required to oxidize water stepwise in aqueous solution (left) and in the OEC of PS II (right). In particular the removal of the first electron from water (left) requires much energy (> 2 eV) that cannot be provided in a biological system. Thus, in the OEC, water is not oxidized by subsequent single electron removal from substrate water. Instead, it is the Mn cluster that is oxidized by four successive oxidation events; the two attached substrate water molecules release the protons (for charge neutrality), and O2 is released only in the last step after O–O bond formation in a concerted reaction. Thereby, high-energy steps are avoided and the redox process is leveled. Figure adapted from (Messinger and Renger 2008)
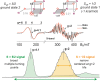
Top: DFT-optimized structures of the Mn4O5Ca cluster in the S2 state (distances given in Ångström). Note that the “closed cubane” (left) and “open cubane” (right) structures have almost the same energy but different positions of the MnIII ion (Mn4 vs. Mn1, respectively) and different total (effective) spin ground states. This explains the two EPR signals observed for this state (shown below as first derivative X-band EPR spectra), with g = 4.1 (Seff = 5/2; no hyperfine structure) and g = 2 (Seff = ½; multiline signal). The bottom trace shows simulations of the two EPR absorption signals. Figure modified from (Krewald et al. ; Pantazis et al. 2012)
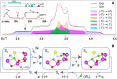
A W-band EPR spectrum of the OEC in the S3 state in PS II of T. elongatus (S3–S1 light–dark difference spectrum) characteristic of an Seff = 3 ground state. The red-dashed line shows a simulation with g and fine structure (zero field splitting) parameters D and E/D; the shaded curves show the contributions of the allowed EPR transitions to the simulated powder spectrum, the color code is indicated. As insert a 55Mn EDNMR spectrum is shown obtained at the EPR position indicated by the arrow. The used EDNMR pulse sequence is given above. Figure modified from (Cox et al. 2014). B Structure of the manganese cluster proceeding through the S2 → S3 → S4 states including water Wf binding, proton release and O2 formation as suggested by magnetic spectroscopy
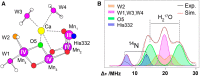
A Mn cluster with the µ-oxo bridges and bound H2O/OH− molecules in the S2 state; the same color code as in panel (B) is used. B17O EDNMR spectrum at W-band (94 GHz) obtained from PS II core preparations (T. elongatus) in the S2 state. The buffer has been exchanged with H217O (90% enrichment) for 30 min (3 ×) in the dark (S1 state) prior to flash-advancement to the S2 state (black trace). EDNMR spectra are also shown for H216O buffer, containing only 14N signals (dark blue trace, 14N from histidine His332, see Fig. 2C). Three classes of oxygen ligands were assigned: the largest 17O splitting belongs to the exchangeable µ-oxo bridge O5 (green), the smaller ones to the H2O/OH− molecules (orange, purple) indicated in the figure. For further details see (Rapatskiy et al. 2012)
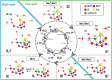
Model for the water oxidation cycle in PS II based on spectroscopic and theoretical work (Cox et al. ; Krewald et al. 2016) detailing the structures of the Mn cluster in the different S states, the water binding events and the O–O bond formation and O2 release in S4. The boxes show the determined oxidation states of the Mn ions in the respective S state. A color code is used for the assignment of the MnIII (light purple) and MnIV (dark purple) ions when the OEC is passing through the S states. Note that the oxygens of the waters and µ-oxo bridges are given in red except for the proposed substrate oxygens O5 and O6 (green). The S2 state exists in two conformations (closed/open cubanes), see text. S3 may also exist in an open (S3A) and a closed (S3B) cubane form (not shown). For S3 a state S3′ is shown in which manganese oxidation has occurred but the 2nd water has not been introduced. This state is present in native sample in a minority of PS II centers, but it is stabilized upon specific chemical treatment (methanol addition or Ca2+ to Sr2+ exchange) (Chrysina et al. 2019). A switching of the preferred total spin ground state configuration of the cluster is thought to take place in S2 from low to high spin and between S4 and S0 back from high to low spin; this is indicated by the diagonal line dividing the green and blue boxes. This switching of the spin state may be necessary for the formation of triplet dioxygen 3O2 in the final step of the cycle (see also Fig. 10). Figure changed from (Krewald et al. 2016)
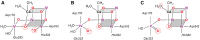
Three representative model structures for the final step in the Kok cycle with O5 and O6 bound (see Figs. 8, 10); A Mn(IV)-oxyl formation at Mn1, octahedral coordination, all Mn(IV), open cubane structure; B Mn(IV)-oxyl formation at Mn4, octahedral, closed cubane structure; C Mn(V) = O formation at Mn4, trigonal bipyramidal, closed cubane. The putative reacting oxygens are indicated by dotted red circles; for details see text. Figure adopted from (Pantazis 2018)
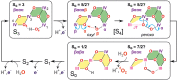
Spin alignment in the S3, [S4] and S0 states that fosters low barrier O–O bond formation as described by Siegbahn (Siegbahn 2009). S3 and S0 have been characterized experimentally; [S4] has not been observed. The spin ground state SG is indicated. The numbering of the Mn ions (1–4) is given for the S3 state; the oxidation state of each Mn ion is given in light purple (III) or dark purple (IV); antiferromagnetic interaction between adjacent Mn ions is represented by yellow shading whereas ferromagnetic interaction by green shading. The spin alignment of the Mn ions in each state is given on the top (the order is: Mn4-Mn3-Mn2-Mn1); the spin of the oxyl radical at the [S4 oxyl] state is indicated by the red colored β. The Jahn–Teller (JT) axis of the MnIII of the [S4 peroxo] intermediate is depicted by a dashed purple line. Evidence of the spin alignment of Mn4-O5-O6-Mn1 in the [S4 oxyl] state (βαβα) is derived from experiments on the S3 state (Cox et al. ; Krewald et al. 2016). For details of the mechanism see text
Similar articles
-
Cox N, Pantazis DA, Lubitz W. Cox N, et al. Annu Rev Biochem. 2020 Jun 20;89:795-820. doi: 10.1146/annurev-biochem-011520-104801. Epub 2020 Mar 24. Annu Rev Biochem. 2020. PMID: 32208765 Review.
-
Cox N, Pantazis DA, Neese F, Lubitz W. Cox N, et al. Acc Chem Res. 2013 Jul 16;46(7):1588-96. doi: 10.1021/ar3003249. Epub 2013 Mar 18. Acc Chem Res. 2013. PMID: 23506074 Review.
-
Water oxidation in oxygenic photosynthesis studied by magnetic resonance techniques.
Lubitz W, Pantazis DA, Cox N. Lubitz W, et al. FEBS Lett. 2023 Jan;597(1):6-29. doi: 10.1002/1873-3468.14543. Epub 2022 Dec 2. FEBS Lett. 2023. PMID: 36409002 Review.
-
Cox N, Retegan M, Neese F, Pantazis DA, Boussac A, Lubitz W. Cox N, et al. Science. 2014 Aug 15;345(6198):804-8. doi: 10.1126/science.1254910. Epub 2014 Aug 14. Science. 2014. PMID: 25124437
Cited by
-
Bicarbonate is a key regulator but not a substrate for O2 evolution in Photosystem II.
Vinyard DJ, Govindjee G. Vinyard DJ, et al. Photosynth Res. 2024 Oct;162(1):93-99. doi: 10.1007/s11120-024-01111-8. Epub 2024 Jul 22. Photosynth Res. 2024. PMID: 39037690 Free PMC article. Review.
-
Magyar M, Sipka G, Han W, Li X, Han G, Shen JR, Lambrev PH, Garab G. Magyar M, et al. Int J Mol Sci. 2022 Dec 21;24(1):94. doi: 10.3390/ijms24010094. Int J Mol Sci. 2022. PMID: 36613535 Free PMC article.
-
The Biochemical Properties of Manganese in Plants.
Schmidt SB, Husted S. Schmidt SB, et al. Plants (Basel). 2019 Sep 27;8(10):381. doi: 10.3390/plants8100381. Plants (Basel). 2019. PMID: 31569811 Free PMC article. Review.
-
Primary donor triplet states of Photosystem I and II studied by Q-band pulse ENDOR spectroscopy.
Niklas J, Agostini A, Carbonera D, Di Valentin M, Lubitz W. Niklas J, et al. Photosynth Res. 2022 May;152(2):213-234. doi: 10.1007/s11120-022-00905-y. Epub 2022 Mar 15. Photosynth Res. 2022. PMID: 35290567 Free PMC article.
-
The Interaction of Water-Soluble Nitroxide Radicals with Photosystem II.
Trubitsin BV, Milanovsky GE, Mamedov MD, Semenov AY, Tikhonov AN. Trubitsin BV, et al. Appl Magn Reson. 2022;53(7-9):1053-1067. doi: 10.1007/s00723-021-01425-z. Epub 2021 Sep 9. Appl Magn Reson. 2022. PMID: 34522067 Free PMC article.
References
-
- acatech—National Academy of Science and Engineering, Leopoldina—German National Academy of Sciences, Union of the German Academies of Sciences and Humanities, editor. Artificial photosynthesis. Munich: National Academy of Science and Engineering; 2018.
-
- Ames W, Pantazis DA, Krewald V, Cox N, Messinger J, Lubitz W, Neese F. Theoretical evaluation of structural models of the S2 state in the oxygen evolving complex of photosystem II: protonation states and magnetic interactions. J Am Chem Soc. 2011;133:19743–19757. doi: 10.1021/ja2041805. - DOI - PubMed
Publication types
MeSH terms
Substances
Supplementary concepts
LinkOut - more resources
Full Text Sources
Other Literature Sources