ABCC9-related Intellectual disability Myopathy Syndrome is a KATP channelopathy with loss-of-function mutations in ABCC9 - PubMed
- ️Tue Jan 01 2019
doi: 10.1038/s41467-019-12428-7.
Conor McClenaghan 2 , Helen I Roessler 3 , Sanne Savelberg 3 , Geir Åsmund Myge Hansen 4 , Helene Hjellnes 4 , Kjell Arne Arntzen 5 6 7 , Kai Ivar Müller 5 6 , Andreas Rosenberger Dybesland 7 8 , Theresa Harter 2 , Monica Sala-Rabanal 2 9 , Chris H Emfinger 2 , Yan Huang 2 10 , Soma S Singareddy 2 , Jamie Gunn 11 , David F Wozniak 11 , Attila Kovacs 12 , Maarten Massink 3 , Federico Tessadori 3 13 , Sarah M Kamel 13 , Jeroen Bakkers 13 14 , Maria S Remedi 15 , Marijke Van Ghelue 4 16 , Colin G Nichols 2 , Gijs van Haaften 17
Affiliations
- PMID: 31575858
- PMCID: PMC6773855
- DOI: 10.1038/s41467-019-12428-7
ABCC9-related Intellectual disability Myopathy Syndrome is a KATP channelopathy with loss-of-function mutations in ABCC9
Marie F Smeland et al. Nat Commun. 2019.
Abstract
Mutations in genes encoding KATP channel subunits have been reported for pancreatic disorders and Cantú syndrome. Here, we report a syndrome in six patients from two families with a consistent phenotype of mild intellectual disability, similar facies, myopathy, and cerebral white matter hyperintensities, with cardiac systolic dysfunction present in the two oldest patients. Patients are homozygous for a splice-site mutation in ABCC9 (c.1320 + 1 G > A), which encodes the sulfonylurea receptor 2 (SUR2) subunit of KATP channels. This mutation results in an in-frame deletion of exon 8, which results in non-functional KATP channels in recombinant assays. SUR2 loss-of-function causes fatigability and cardiac dysfunction in mice, and reduced activity, cardiac dysfunction and ventricular enlargement in zebrafish. We term this channelopathy resulting from loss-of-function of SUR2-containing KATP channels ABCC9-related Intellectual disability Myopathy Syndrome (AIMS). The phenotype differs from Cantú syndrome, which is caused by gain-of-function ABCC9 mutations, reflecting the opposing consequences of KATP loss- versus gain-of-function.
Conflict of interest statement
The authors declare no competing interests.
Figures
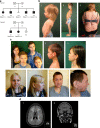
Clinical features and pedigrees of AIMS patients. a Pedigrees of both affected families. Black defines patients homozygous for the ABCC9 c.1320 + 1 G < A mutation. Black dots indicate individuals heterozygous for the ABCC9 variant. Gray triangle represents a fetus affected by probable thanatophoric dysplasia and terminated pregnancy. b Musculoskeletal features in AIMS patients. (1) Lumbar lordosis in patient 1–4 at age 4; (2) lumbar lordosis, thin habitus in patient 1–2 at age 10; (3) thoracolumbar scoliosis in patient 2–2 at age 28. c Facial features with prominent orbital ridges, hypotelorism, thin upper lip, flat midface in several of the patients. (1) Family 1. Upper left: patient 1–1 at age 20. Upper right: patient 1–2 at age 16. Lower left: patient 1–3 at age 11. Lower right: patient 1–4 at age 10; (2) profile of patient 1–2 at age 10; (3) profile of patient 1–1 at age 15; (4) patient 2–2 at age 28; (5) patient 2–1 at age 32. d Cerebral MRI of AIMS patients. (1) Magnetic resonance imaging (MRI) of the brain of patient 2–1. T2-weighted fluid-attenuated inversion recovery (FLAIR), coronal section shows widespread white matter hyperintensities. (2) MRI of the brain of patient 1–1. T2-weighted FLAIR, axial section shows juxtacortical white matter hyperintensities
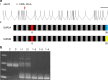
Molecular analysis in AIMS patients. a Genomic organization of the ABCC9 gene: (1) basic genomic structure of ABCC9 includes at least 39 potential exons, excluding untranslated regions (UTRs). The c.1320 + 1 G > A mutation predicted to disrupt the splice donor site of exon 8 is indicated by a red arrow. Scale bar, 5000 bp. (2) The mutation impacts both the SUR2A and SUR2B splice forms, which differ only in the last exon. Affected exon 8 in patients is marked in red. Odd-numbered exons are presented as black boxes, even-numbered exons as gray boxes. b Analysis of the effect of the mutation at the cDNA level in Family 1. Two control cDNA samples (indicated by a C) show the wild-type PCR product containing exon 8, parents (I:1, I:2) show heterozygosity for the wt and a lower band lacking exon 8, whereas patient cDNA (1–2, 1–3, 1–4) only yielded the lower band
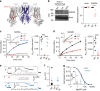
Exon 8 deletion results in KATP channel loss-of-function. a KATP channel structure showing pore-forming Kir6.x subunits (blue) associated with two of four SUR subunits (gray) (PDB 5WUA [doi 10.2210/pdb5WUA/pdb]), with the equivalent position of the SUR2 amino acids encoded by ABCC9 exon 8 in red (Ala389-Gln440). b Western blot of whole-cell lysate from Cosm6 cells transiently transfected with GFP or Kir6.2 alongside either wild-type SUR2A-Flag (WT) or SUR2A-Flag∆8. Left: Immunoblots using a primary antibody targeting the Flag-tag of SUR2A-Flag∆8 (top) and actin control (bottom). Right: Normalized expression for actin and SUR2A-Flag from cells transfected with WT SUR2A-Flag or SUR2A-Flag∆8. The data from four independent experiments, * denotes p < 0.05 according to Mann–Whitney U Test. A representative example blot is included in the associated Source Data File. c Left: 86Rb+ efflux from Cosm6 cells transfected with GFP alone, Kir6.2 alongside WT SUR2A, SUR2A∆8, or a 1:1 ratio of WT SUR2A and SUR2A∆8 (WT:∆8). Inset: early time efflux/time data points used to derive efflux rate constants. Right: Efflux rate constants for cells transfected with WT SUR2A, SUR2A∆8, and 1:1 ratio of WT:∆8. The data from six replicates in three independent experiments, ****p < 0.0001 (Mann–Whitney U Test). d Left: 86Rb+ efflux experiment from Cosm6 cells transfected with GFP alone, with Kir6.2 alongside WT SUR2B or SUR2B∆8. Right: Efflux rate constants for cells transfected with WT SUR2B or SUR2B∆8. The data from six replicates in three independent experiments, ****p < 0.0001 (Mann–Whitney U Test). e Example inside–out patch clamp recordings from Cosm6 cells transfected with Kir6.2 alongside SUR2A-WT (black), SUR2A∆8 (red), or a 1:1 mix of SUR2A-WT and SUR2A∆8 to mimic heterozygous expression (blue). The membrane potential was held at −50 mV in symmetrical KINT solutions, and ATP was applied as indicated. Arrows indicate the point of patch excision. f KATP currents from excised patches. g ATP dose–response relationship for SUR2A-WT or 1:1 SUR2A-WT:SUR2A∆8 channels. Inset: summary of ATP IC50 values. The data from individual experiments shown as dots alongside mean ± SEM. Source data are provided as a Source Data file
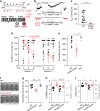
SUR2-STOP mice exhibit cardiac dysfunction and fatigability. a Top: The c.3446_3450delACTTCinsGA indel in ABCC9 and consequent premature stop codon following K1148 (p.Y1149Stop). Bottom: schematic of SUR2 with the site of the introduced Y1149Stop mutation in TM15 indicated and the downstream region in red. b Example current traces from inside–out voltage clamp recordings from ventricular myocytes of WT (black) or SUR2-STOP (red) mice (−50 mV holding potential in the presence and absence of MgATP and pinacidil as indicated). Scale bar shows 5 s (x-axis) and 25 pA (y-axis). c KATP channel current amplitudes from excised patches from mouse ventricular myocytes. The data shown from 18 patches for WT, and 10 patches for SUR2-STOP from ≥ 3 mice. ****p < 0.0001 (two-tailed t test). d Duration mice remained inverted during the multiple-trial inverted screen test. The data were analyzed using a repeated measures (rm) ANOVA model that contained one between-subjects variable (genotype) and two within-subjects variables (trials and sessions; see Supplementary Table 3 for summary statistics). The results from the rmANOVA revealed a significant genotype effect, as well as genotype x trial and genotype x session interactions. The data from nine WT and nine SUR2-STOP mice, *p-values for the pairwise comparisons exceeded Bonferroni correction (p < 0.008 [0.05/6]; *p < 0.05; **p < 0.01; ***p < 0.001). e) Cumulative inversion time, *p < 0.05 and **p < 0.01 according to one-way ANOVA and post hoc Tukey test. f Example M-mode echocardiography recordings from WT (top) and SUR2-STOP (bottom) mice. Scale bar shows 0.1 s (x-axis) and 1 mm (y-axis). g Ventricular fractional shortening measured from echocardiographic imaging (all echocardiographic data from five WT and five SUR2-STOP mice), **p < 0.01 (one-way ANOVA and post hoc Tukey test). h Left ventricular mass (LVM) as determined from echocardiography imaging normalized to body (LVM/BW) and body length (LVM/BL). *p < 0.05 (student’s t test). i Left ventricular internal diameter in diastole as measured from echocardiographic imaging. *p < 0.05 (student’s t test). The data from individual experiments shown as dots alongside mean ± SEM. Source data are provided as a Source Data file
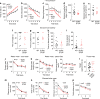
No major behavioral or cognitive deficits observed in SUR2-STOP mice. a Total time at rest b The total number of ambulations (whole-body movements), c the number of incidents of rearing, d distance traveled at edge of the observational field, and e distance traveled within center of observational field in 1 h locomotion observation, n = 11 for WT and 9 for SUR2-STOP for all behavioral tests. f Time mice spent on elevated ledge, g time mice remained on elevated circular platform, h time taken to descend during the pole test, and i time taken to ascend a wire-mesh screen maintained at 60o angle for WT and SUR2-STOP mice in sensorimotor battery. j The escape path length taken to find the platform by WT and SUR2-STOP mice in Morris Water Maze Cued trials. Note, no significant difference in swimming speed was observed between genotypes. k The mean escape path length (left) taken to find the platform, and average swimming speed (right) by WT and SUR2-STOP mice in Morris Water Maze Place trials. l The time spent within the target quadrant (the quadrant in which the platform had previously been positioned during Cued and Place trials) was measured. m Distance traveled within open arms, (n) time spent in open arms, o entries into open arms, and p total distance traveled in the Elevated Plus Maze test for WT and SUR2-STOP mice. The data were analyzed using a repeated measures (rm) ANOVA model that contained two between-subjects variable (genotype and sex) and one within-subjects variable (trial block). The data from individual experiments shown as dots alongside mean ± SEM. Source data are provided as a Source Data file
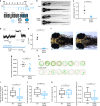
Hypotolerism and decreased locomotor behavior in SUR2-STOP zebrafish larvae. a The c.2944_2957del13 indel in abcc9 and consequent frameshift premature stop codon following S984 (p.S985Stop) and schematic of SUR2 with the site of the introduced S985Stop mutation in TM12 indicated, downstream region in blue. b Representative images illustrating the morphology of 5 dpf wild-type and SUR2-STOP mutants as seen from a left lateral (top) and dorsal view (bottom). Scale bars, 1 mm. c Quantitative RT-PCR to assess abcc9 expression in pools of 60 WT (three pools) and SUR2-STOP (four pools) embryos. d Representative current traces from inside–out patch clamp recordings from ventricular myocytes of WT (black; five patches) or SUR2-STOP (blue; six patches) zebrafish (−50 mV holding potential, ATP applied as indicated). e KATP channel currents from excised patches from zebrafish ventricular myocytes. The data from five patches (WT), and six patches (SUR2-STOP) from ≥ 3 zebrafish. f, g To assess hypotelorism, the distance between the convex tips of the eyes was measured and normalized to body length (WT, n = 8; HOM, n = 10). Scale bars, 200 μm. h Examples of movement traces shown in red, green, and black representing high-speed, intermediate, and slow movements, respectively. i–l Five-minute video recordings (n = 62 larvae per genotype) of 5 dpf homozygous SUR2-STOP fish and wild-type controls were analyzed for total amount of movement i, total swimming distance (TSD) j, total swimming duration k, and duration of high-speed movements l and compared with respective wild-type larvae. The data on the y-axis refer to the respective average value per 30-s (s) intervals. The data from four independent experiments (16 larvae per experiment) *p ≤ 0.05; **p ≤ 0.01; ***p ≤ 0.001; ****p ≤ 0.0001 (two-tailed unpaired Student’s t test or Mann–Whitney U test). The black horizontal bar indicates the mean value for each condition. Sample size, WT, n = 3; SUR2-STOP, n = 4 in c; WT, n = 5; SUR2-STOP, n = 5 in e; WT, n = 8; SUR2-STOP, n = 10 in g; WT, n = 62; SUR2-STOP, n = 62 in i–l. The data from individual experiments shown as dots alongside mean ± SEM. Source data are provided as a Source Data file
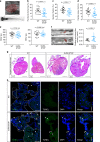
Systolic dysfunction and enlarged heart size in SUR2-STOP zebrafish. a Box designates imaged area to assess cardiac function. The ventricular area of the heart is highlighted, with the long axis and short axis of the ventricle indicated by dashed lines. b–e Quantification of cardiac function using individual characteristic confocal sections from a time series of the embryonic cardiac cycle at 5 dpf. f Tracking of individual red blood cells (RBCs) measuring cardinal vein blood flow velocity. RBCs were tracked for ten frames using ImageJ (NIH) and the plugin MTrackJ. One representative image of each genotype is shown. Black arrow indicates the direction of RBC movement. g Heart histology of adult SUR2-STOP mutants and respective siblings after H&E staining. Exemplary depiction of 2 WT and 2 SUR2-STOP hearts. For assessment of ventricular chamber size, tissue sections showing the largest ventricular area were selected. h TUNEL assay on adult hearts to detect apoptotic cells (white arrowheads) in WT and SUR2-STOP fish. Heart chambers are indicated by white dashed line. Nuclei are stained with DAPI. All experiments were performed comparing SUR2-STOP and its WT siblings. For all graphs, significance was determined by two-tailed unpaired Student’s t test or Mann–Whitney two-tailed U test. Asterisks indicate statistical significance (*p ≤ 0.05; **p ≤ 0.01; ***p ≤ 0.001; ****p ≤ 0.0001). The black horizontal bar indicates the mean value for each condition. Sample size, WT, n = 20; SUR2-STOP, n = 20 in b–e; WT, n = 14; SUR2-STOP, n = 21 in f; WT, n = 6; SUR2-STOP, n = 6 in g and h. Scale bars, 1 mm and 50 μm in a; 10 μm in f, 500 μm in g; 100 μm (overview) and 50 μm (close up) in h. All embryos analyzed originated from group matings of adult zebrafish. a atrium, ba bulbous arteriosus, v ventricle. The data from individual experiments shown as dots alongside mean ± SEM. Source data are provided as a Source Data file
Similar articles
-
Cooper PE, Sala-Rabanal M, Lee SJ, Nichols CG. Cooper PE, et al. J Gen Physiol. 2015 Dec;146(6):527-40. doi: 10.1085/jgp.201511495. J Gen Physiol. 2015. PMID: 26621776 Free PMC article.
-
Novel loss-of-function variants expand ABCC9-related intellectual disability and myopathy syndrome.
Efthymiou S, Scala M, Nagaraj V, Ochenkowska K, Komdeur FL, Liang RA, Abdel-Hamid MS, Sultan T, Barøy T, Van Ghelue M, Vona B, Maroofian R, Zafar F, Alkuraya FS, Zaki MS, Severino M, Duru KC, Tryon RC, Brauteset LV, Ansari M, Hamilton M, van Haelst MM, van Haaften G, Zara F, Houlden H, Samarut É, Nichols CG, Smeland MF, McClenaghan C. Efthymiou S, et al. Brain. 2024 May 3;147(5):1822-1836. doi: 10.1093/brain/awae010. Brain. 2024. PMID: 38217872 Free PMC article.
-
McClenaghan C, Hanson A, Sala-Rabanal M, Roessler HI, Josifova D, Grange DK, van Haaften G, Nichols CG. McClenaghan C, et al. J Biol Chem. 2018 Feb 9;293(6):2041-2052. doi: 10.1074/jbc.RA117.000351. Epub 2017 Dec 22. J Biol Chem. 2018. PMID: 29275331 Free PMC article.
-
Nelson PT, Jicha GA, Wang WX, Ighodaro E, Artiushin S, Nichols CG, Fardo DW. Nelson PT, et al. Ageing Res Rev. 2015 Nov;24(Pt B):111-25. doi: 10.1016/j.arr.2015.07.007. Epub 2015 Jul 28. Ageing Res Rev. 2015. PMID: 26226329 Free PMC article. Review.
-
[A new type of ATP-sensitive potassium channelopathy : Cantú syndrome].
Hiraki Y, Takanari H. Hiraki Y, et al. No To Hattatsu. 2016 Sep;48(5):325-31. No To Hattatsu. 2016. PMID: 30010274 Review. Japanese.
Cited by
-
Li Z, Fan Q, Chen M, Dong Y, Li F, Wang M, Gu Y, Guo S, Ye X, Wu J, Dai S, Lin R, Zhao C. Li Z, et al. J Pharm Anal. 2023 Jan;13(1):39-54. doi: 10.1016/j.jpha.2022.11.005. Epub 2022 Nov 19. J Pharm Anal. 2023. PMID: 36820075 Free PMC article.
-
Skeletal muscle delimited myopathy and verapamil toxicity in SUR2 mutant mouse models of AIMS.
McClenaghan C, Mukadam MA, Roeglin J, Tryon RC, Grabner M, Dayal A, Meyer GA, Nichols CG. McClenaghan C, et al. EMBO Mol Med. 2023 Jun 7;15(6):e16883. doi: 10.15252/emmm.202216883. Epub 2023 May 8. EMBO Mol Med. 2023. PMID: 37154692 Free PMC article.
-
Transcriptome-Guided Identification of Drugs for Repurposing to Treat Age-Related Hearing Loss.
Schubert NMA, van Tuinen M, Pyott SJ. Schubert NMA, et al. Biomolecules. 2022 Mar 25;12(4):498. doi: 10.3390/biom12040498. Biomolecules. 2022. PMID: 35454087 Free PMC article.
-
Gauthreaux KM, Teylan MA, Katsumata Y, Mock C, Culhane JE, Chen YC, Chan KCG, Fardo DW, Dugan AJ, Cykowski MD, Jicha GA, Kukull WA, Nelson PT. Gauthreaux KM, et al. Neurology. 2022 Apr 5;98(14):e1422-e1433. doi: 10.1212/WNL.0000000000200001. Epub 2022 Feb 4. Neurology. 2022. PMID: 35121671 Free PMC article.
-
Case Report: Loss-of-Function ABCC9 Genetic Variant Associated With Ventricular Fibrillation.
Zaytseva A, Tulintseva T, Fomicheva Y, Mikhailova V, Treshkur T, Kostareva A. Zaytseva A, et al. Front Genet. 2022 Apr 13;13:718853. doi: 10.3389/fgene.2022.718853. eCollection 2022. Front Genet. 2022. PMID: 35495129 Free PMC article.
References
Publication types
MeSH terms
Substances
Supplementary concepts
LinkOut - more resources
Full Text Sources
Medical
Molecular Biology Databases
Miscellaneous