Spider Venom: Components, Modes of Action, and Novel Strategies in Transcriptomic and Proteomic Analyses - PubMed
- ️Tue Jan 01 2019
Review
Spider Venom: Components, Modes of Action, and Novel Strategies in Transcriptomic and Proteomic Analyses
Nicolas Langenegger et al. Toxins (Basel). 2019.
Abstract
This review gives an overview on the development of research on spider venoms with a focus on structure and function of venom components and techniques of analysis. Major venom component groups are small molecular mass compounds, antimicrobial (also called cytolytic, or cationic) peptides (only in some spider families), cysteine-rich (neurotoxic) peptides, and enzymes and proteins. Cysteine-rich peptides are reviewed with respect to various structural motifs, their targets (ion channels, membrane receptors), nomenclature, and molecular binding. We further describe the latest findings concerning the maturation of antimicrobial, and cysteine-rich peptides that are in most known cases expressed as propeptide-containing precursors. Today, venom research, increasingly employs transcriptomic and mass spectrometric techniques. Pros and cons of venom gland transcriptome analysis with Sanger, 454, and Illumina sequencing are discussed and an overview on so far published transcriptome studies is given. In this respect, we also discuss the only recently described cross contamination arising from multiplexing in Illumina sequencing and its possible impacts on venom studies. High throughput mass spectrometric analysis of venom proteomes (bottom-up, top-down) are reviewed.
Keywords: Araneae; bioinformatics; mass spectrometry; neurotoxins; proteomics; spiders; transcriptomics; venomics.
Conflict of interest statement
The authors declare no conflict of interest.
Figures
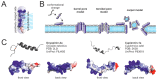
Antimicrobial peptides (AMPs) and their proposed mechanism of action. (A) Model of interaction between an AMP and phospholipids. AMPs assume an amphipathic α-helical structure in proximity to cellular membranes. The hydrophobic side of the helix (white) inserts into the membrane and interacts with the phospholipid side chains. The positively charged side (blue) interacts with negatively charged lipid head groups. (B) Models of membranolytic actions of AMPs. (C) NMR-based 3D structures of two antimicrobial peptides from spider venom. Electrostatics were computed using PDB2PQR [70]. Blue surfaces represent positively charged surfaces; red negative charged; and white neutral.
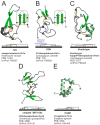
Structural motifs of cysteine-rich spider venom peptides. Disulphide connected cysteines are numbered and disulphide bridges are shown in yellow. A linear schematic representation of the disulphide bridge pattern is shown below the corresponding 3D structure. (A) Inhibitor cystine knot motif. The disulphide bridge colored in orange penetrates the ring opened by the peptide backbone and the two other disulphide bridges. (B) Disulphide-directed β-hairpin motif. The disulphide bridge colored in blue is optional for this motif. (C) Kunitz-type motif. (D) Colipase or MIT1-like motif. (E) Helical arthropod-neuropeptide-derived motif.
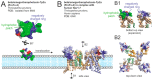
Binding of neurotoxins to a voltage gated ion channel. Peptides are shown space filling, ion channels as cartoons with every domain in a different color. (A) Hydrophobic patch with surrounding ring of charged amino acids. Hydrophobic amino acids are shown in green, negatively charged in blue, and positively charged in red. (B) Four ProTx-2 toxins bound to the human NaV1.7 channel in side view. Toxin surface represents surface charge. Eye-icons and arrows indicate the viewing angel in panels B1 and B2. (B1) View on one voltage-sensing domain of the channel bound to the toxin from the inner side of the membrane towards outwards. (B2) View on the toxin-channel complex form the top. Helices of the voltage sensing domain are indicated with S1–S4.
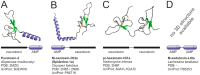
3D structures and schematic structures of modular toxins (multi-domain toxins). Antimicrobial domains are highlighted in blue, disulphide bridges in yellow. (A) Neurotoxin-AMP. (B) AMP-neurotoxin. The structure of spiderine-1a is assembled from two experimental 3D structures of the N- and C-terminal toxin part, respectively. (C) Neurotoxin-neurotoxin. (D) AMP-AMP.
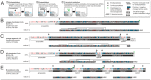
Maturing of venom peptides. (A) Stepwise schematic representation of the general maturing processes on the example of CsTx-13 (see panel D). (1) The Processing Quadruplet Motif (PQM) protease cleaves C-terminal of the Arg residue of the PQM or inverted Processing Quadruplet Motif (iPQM). (2) A so far uncharacterized carboxypeptidase subsequently removes the C-terminally exposed Arg if present, and (3) if a C-terminally exposed Gly is present, this is eliminated under formation of a C-terminal amide. (B–E) Schematic representation of chosen precursor sequences (top) and the corresponding mature sequences of neurotoxins. Maturing processes are indicated by arrows and triangles (see legend in the top right corner). (B) Precursor of a monomeric neurotoxin. (C,D) Precursors of heterodimeric neurotoxins. (E) N-terminal segment of a complex AMP precursor. Amino acids with positive side-chain charges at pH 7 are shown in blue, such with negative charges at pH 7 in red. All here shown precursors also comprise signal peptides which are not shown for reasons of space.
Similar articles
-
Pineda SS, Chin YK, Undheim EAB, Senff S, Mobli M, Dauly C, Escoubas P, Nicholson GM, Kaas Q, Guo S, Herzig V, Mattick JS, King GF. Pineda SS, et al. Proc Natl Acad Sci U S A. 2020 May 26;117(21):11399-11408. doi: 10.1073/pnas.1914536117. Epub 2020 May 12. Proc Natl Acad Sci U S A. 2020. PMID: 32398368 Free PMC article.
-
Proteotranscriptomic Insights into the Venom Composition of the Wolf Spider Lycosa tarantula.
Koua D, Mary R, Ebou A, Barrachina C, El Koulali K, Cazals G, Charnet P, Dutertre S. Koua D, et al. Toxins (Basel). 2020 Aug 5;12(8):501. doi: 10.3390/toxins12080501. Toxins (Basel). 2020. PMID: 32764230 Free PMC article.
-
Kuhn-Nentwig L. Kuhn-Nentwig L. Sci Rep. 2021 Feb 17;11(1):4009. doi: 10.1038/s41598-021-83624-z. Sci Rep. 2021. PMID: 33597701 Free PMC article.
-
Proteome and peptidome profiling of spider venoms.
Liang S. Liang S. Expert Rev Proteomics. 2008 Oct;5(5):731-46. doi: 10.1586/14789450.5.5.731. Expert Rev Proteomics. 2008. PMID: 18937563 Review.
-
Scorpion and spider venom peptides: gene cloning and peptide expression.
Quintero-Hernández V, Ortiz E, Rendón-Anaya M, Schwartz EF, Becerril B, Corzo G, Possani LD. Quintero-Hernández V, et al. Toxicon. 2011 Dec 1;58(8):644-63. doi: 10.1016/j.toxicon.2011.09.015. Epub 2011 Sep 28. Toxicon. 2011. PMID: 21978889 Review.
Cited by
-
Venom-gland transcriptomics and venom proteomics of the Tibellus oblongus spider.
Korolkova Y, Mikov A, Lobas A, Solovyeva E, Surin A, Andreev Y, Gorshkov M, Kozlov S. Korolkova Y, et al. Sci Data. 2023 Nov 22;10(1):820. doi: 10.1038/s41597-023-02703-0. Sci Data. 2023. PMID: 37993463 Free PMC article.
-
The Deadly Toxin Arsenal of the Tree-Dwelling Australian Funnel-Web Spiders.
Cardoso FC, Pineda SS, Herzig V, Sunagar K, Shaikh NY, Jin AH, King GF, Alewood PF, Lewis RJ, Dutertre S. Cardoso FC, et al. Int J Mol Sci. 2022 Oct 28;23(21):13077. doi: 10.3390/ijms232113077. Int J Mol Sci. 2022. PMID: 36361863 Free PMC article.
-
Rojas-Palomino J, Gómez-Restrepo A, Salinas-Restrepo C, Segura C, Giraldo MA, Calderón JC. Rojas-Palomino J, et al. J Venom Anim Toxins Incl Trop Dis. 2024 Sep 2;30:e20230048. doi: 10.1590/1678-9199-JVATITD-2023-0048. eCollection 2024. J Venom Anim Toxins Incl Trop Dis. 2024. PMID: 39263598 Free PMC article.
-
Ahmed J, Walker AA, Perdomo HD, Guo S, Nixon SA, Vetter I, Okoh HI, Shehu DM, Shuaibu MN, Ndams IS, King GF, Herzig V. Ahmed J, et al. Toxins (Basel). 2023 Jun 27;15(7):418. doi: 10.3390/toxins15070418. Toxins (Basel). 2023. PMID: 37505687 Free PMC article.
-
Richards NJ, Alqallaf A, Mitchell RD, Parnell A, Haidar HB, Almeida JR, Williams J, Vijayakumar P, Balogun A, Matsakas A, Trim SA, Patel K, Vaiyapuri S. Richards NJ, et al. Cells. 2023 Aug 15;12(16):2074. doi: 10.3390/cells12162074. Cells. 2023. PMID: 37626884 Free PMC article.
References
Publication types
MeSH terms
Substances
LinkOut - more resources
Full Text Sources
Other Literature Sources
Miscellaneous