ELAVL2-directed RNA regulatory network drives the formation of quiescent primordial follicles - PubMed
- ️Tue Jan 01 2019
ELAVL2-directed RNA regulatory network drives the formation of quiescent primordial follicles
Yuzuru Kato et al. EMBO Rep. 2019.
Abstract
Formation of primordial follicles is a fundamental, early process in mammalian oogenesis. However, little is known about the underlying mechanisms. We herein report that the RNA-binding proteins ELAVL2 and DDX6 are indispensable for the formation of quiescent primordial follicles in mouse ovaries. We show that Elavl2 knockout females are infertile due to defective primordial follicle formation. ELAVL2 associates with mRNAs encoding components of P-bodies (cytoplasmic RNP granules involved in the decay and storage of RNA) and directs the assembly of P-body-like granules by promoting the translation of DDX6 in oocytes prior to the formation of primordial follicles. Deletion of Ddx6 disturbs the assembly of P-body-like granules and severely impairs the formation of primordial follicles, indicating the potential importance of P-body-like granules in the formation of primordial follicles. Furthermore, Ddx6-deficient oocytes are abnormally enlarged due to misregulated PI3K-AKT signaling. Our data reveal that an ELAVL2-directed post-transcriptional network is essential for the formation of quiescent primordial follicles.
Keywords: DDX6; ELAVL2; P-body-like granules; post-transcriptional gene regulation; primordial follicles.
© 2019 The Authors.
Conflict of interest statement
The authors declare that they have no conflict of interest.
Figures
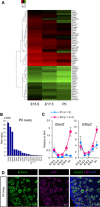
Heat map of genes encoding RNA‐binding proteins (n = 3 independent ovary samples at each developmental stage). Genes whose expression levels were increased by more than twofold in ovaries between E15.5 and P0 are listed. Red and green indicate high‐ and low‐processed signals, respectively.
Genes whose expression increased by more than twofold in ovaries between E17.5 and P0 were selected from 55 gene probes in (A).
Reverse transcription and quantitative polymerase chain reaction (RT–qPCR) analysis of Elavl2 and G3bp2 in XX and XY gonads from E12.5 to P1 (n = 3–5 animals). Error bars, ±SD.
Immunostaining of ELAVL2 in P0 ovaries (n = 3 animals). c‐KIT was used as a germ cell marker. DNA was counterstained with 4′,6‐diamidino‐2‐phenylindole (DAPI).
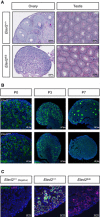
Periodic acid–Schiff (PAS) staining in wild‐type (Elavl2 +/+) and Elavl2 knockout (Elavl2 Δ/Δ) adult ovaries (left) and testes (right) (n = 3 animals for each genotype).
Immunostaining of a germ cell marker, MVH/DDX4, in neonatal ovaries (n = 3 animals for each genotype). DNA was counterstained with 4′,6‐diamidino‐2‐phenylindole (DAPI).
Immunostaining of ELAVL2 newborn ovaries (n = 2 animals for each genotype). Negative; negative control.
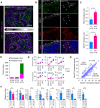
Immunostaining of an extracellular matrix protein, LAMININ, and a germ cell marker, c‐KIT in wild‐type (Elavl2 +/+) and Elavl2 knockout (Elavl2 Δ/Δ) newborn ovaries (n = 3 animals for each genotype).
Immunostaining of TEX14 and MKLP1 in newborn ovaries (n = 3 animals for each genotype). Oocytes were marked by DAZL. Scale bars, 50 μm.
Quantification of TEX14 and MKLP1 foci (n = 3 animals) in (B).
Microarray analysis of wild‐type (WT) and Elavl2 knockout ovaries (n = 3 independent ovary samples). Gene probes up‐ or down‐regulated by more than twofold in Elavl2 knockout ovaries are shown.
Expression profile of selected genes involved in follicular and early zygotic development in (D).
Reverse transcription and quantitative polymerase chain reaction (RT–qPCR) of selected genes (n = 5–7 animals).
Scatter plot analysis of gene probes whose expression increased by more than twofold from E17.5 to P0 in WT ovaries in (D). Probes demonstrating a greater than 1.5‐fold difference between wild‐type and Elavl2 knockout ovaries are shown.
Data information: (C, F, and G) Circles indicate individual data. Error bars, ±SD. The significance is indicated (two‐tailed Student's t‐test; ***P < 0.0005, **P < 0.005, *P < 0.05).
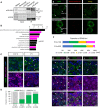
- A
RNA immunoprecipitation of ELAVL2 using WT newborn ovaries. Immunoprecipitated ELAVL2 was confirmed by Western blotting. MVH was used as a negative control (n = 3 independent ovary samples).
- B
Gene ontology analysis (DAVID ver. 6.8) of ELAVL2‐associating mRNAs.
- C
Immunostaining of DDX6 in E17.5 (n = 3 animals) and P1 (n = 10 animals) ovaries. DAZL was used as an oocyte marker.
- D
Quantification of oocytes containing DDX6 foci (n = number of oocytes from two animals at each stage).
- E
Images analysis of DDX6 foci. Images of DDX6 from P0 and P2 ovaries (left) were used to create masks of DDX6 foci (middle). Merged images are shown on the right.
- F
Quantification of DDX6 foci (n = number of oocytes from two animals at each stage).
- G–I
Immunostaining of DCP1A (G) (n = 6 animals), AGO2 (H) (n = 3 animals), and TIAR (I) (n = 5 animals) together with DDX6 in newborn ovaries. DNA was counterstained with DAPI.
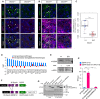
- A, B
Immunostaining of DDX6 together with DCP1A (A) (n = 6 animals for each genotype) and AGO2 (B) (n = 3 animals for each genotype) in wild‐type (Elavl2 +/+) and Elavl2 knockout (Elavl2 Δ/Δ) newborn ovaries.
- C
Quantification of the area of DDX6 foci in newborn ovaries. Twenty (wild‐type) and 21 (Elavk2 knockout) ovarian sections from two animals were immuno‐stained by anti‐DDX6 antibody and analyzed.
- D
Expression changes of mRNAs encoding components of P‐bodies. Blue bars, fold enrichment of ELAVL2‐associating mRNAs in Fig 3B. Red bars, fold expression changes of cognate mRNAs by microarray (P0 ovaries).
- E
Western blotting of DDX6 in newborn ovaries. The expression level of DDX6 in Elavl2 knockout ovaries was normalized by MVH and represented as a ratio to WT.
- F
Co‐transfection assay of full‐length and mutant ELAVL2 with gfp reporters in HeLa cells.
- G
Detection of immunoprecipitated ELAVL2 by Western blotting. ACTB was used as a negative control.
- H
RT–qPCR analysis of gfp reporters. The vertical axis indicates relative quantity of immunoprecipitated gfp to inputs (n = number of experiment).
Data information: (C and H) Circles represent individual data. Error bars, ±SD. Boxes and horizontal bands in boxes represent quartile deviations and median, respectively. The significance of changes is indicated (Wilcoxon rank sum test in (C) and two‐tailed Student's t‐test in (H); ***P < 0.0005, **P < 0.005).
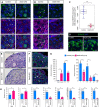
- A, B
Immunostaining of DDX6 together with DCP1A (A) (n = 3 animals for each genotype) and AGO2 (B) (n = 3 animals for each genotype) in newborn ovaries. DNA was counterstained with DAPI.
- C
Quantification of the area of DCP1A foci in newborn ovaries. Twenty‐eight (control) and 25 (Ddx6 cKO) ovarian sections from two animals were immuno‐stained by anti‐DCP1A antibody and analyzed.
- D
Immunostaining of ELAVL2 in newborn ovaries (n = 3 animals for each genotype).
- E
PAS staining of P7 ovaries (n = 5 animals for each genotype).
- F
Immunostaining of an oocyte marker, CDH1, together with LAMININ in P7 ovaries (n = 3 animals for each genotype).
- G
Oocyte counting analysis of P7 ovaries (n = animals). Cyst, oocyte in cyst; PrF, primordial follicle; T, transition; PF, primary follicle; SF, secondary follicle.
- H
RT–qPCR analysis of P7 ovaries (n = 4 animals).
Data information: (C, G, and H) Circles indicate individual data. Error bars, ±SD. Boxes and horizontal bands in boxes represent quartile deviations and median, respectively. The significance of changes is indicated (Wilcoxon rank sum test in (C) and two‐tailed Student's t‐test in (G and H); ***P < 0.0005, **P < 0.005, *P < 0.005).
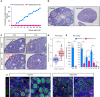
Litter size analysis of Ddx6 cKO females. Ddx6 +/+ , Ddx6 +/+ ; Mvh‐Cre, and Ddx6 flox/+ females were used as control. Control and Ddx6 cKO females (n = 6 animals for each genotype) were crossed with wild‐type males from 6 to 24 weeks after birth.
PAS staining of adult Ddx6 cKO ovaries (n = 6 animals for each genotype).
PAS staining of P14 (n = 3 animals) and 21 (n = 7 animals) ovaries. Areas indicated by white squares are magnified. T, transition; PF, primary follicle; SF, secondary follicle; AF, antral follicle.
Quantification of the oocyte diameter for primordial follicles in P14 ovaries. Data were obtained from two animals for each genotype.
Oocyte counting analysis for P21 ovaries (n = 7 animals for each genotype). T, PF, and SF are the same as in Fig 5G. AF, antral follicle.
Immunostaining of FOXO3A and CDH1 in P1 and 7 ovaries (n = 3 animals for each genotype). PrF, primordial follicle.
Data information: (D and E) Circles indicate individual data. Error bars, ±SD. Boxes and horizontal bands in boxes represent quartile deviations and median, respectively. The significance of changes is indicated (Wilcoxon rank sum test in (D) and two‐tailed Student's t‐test in (E); ***P < 0.0005, **P < 0.005).
Similar articles
-
Kato Y, Saga Y. Kato Y, et al. Biol Reprod. 2023 Jul 11;109(1):73-82. doi: 10.1093/biolre/ioad043. Biol Reprod. 2023. PMID: 37067907
-
Chalupnikova K, Solc P, Sulimenko V, Sedlacek R, Svoboda P. Chalupnikova K, et al. Cell Cycle. 2014;13(7):1187-200. doi: 10.4161/cc.28107. Epub 2014 Feb 11. Cell Cycle. 2014. PMID: 24553115 Free PMC article.
-
Dong MZ, Ouyang YC, Gao SC, Gu LJ, Guo JN, Sun SM, Wang ZB, Sun QY. Dong MZ, et al. Cell Death Dis. 2024 Sep 8;15(9):658. doi: 10.1038/s41419-024-07051-4. Cell Death Dis. 2024. PMID: 39245708 Free PMC article.
-
Growth of primordial oocytes in neonatal and adult mammals.
Moniruzzaman M, Miyano T. Moniruzzaman M, et al. J Reprod Dev. 2010 Dec;56(6):559-66. doi: 10.1262/jrd.10-071h. J Reprod Dev. 2010. PMID: 21245654 Review.
-
Zhang H, Liu K. Zhang H, et al. Hum Reprod Update. 2015 Nov-Dec;21(6):779-86. doi: 10.1093/humupd/dmv037. Epub 2015 Jul 30. Hum Reprod Update. 2015. PMID: 26231759 Review.
Cited by
-
Two mechanisms repress cyclin B1 translation to maintain prophase arrest in mouse oocytes.
Cheng S, Schuh M. Cheng S, et al. Nat Commun. 2024 Nov 20;15(1):10044. doi: 10.1038/s41467-024-54161-w. Nat Commun. 2024. PMID: 39567493 Free PMC article.
-
The molecular genetics of nELAVL in brain development and disease.
Mulligan MR, Bicknell LS. Mulligan MR, et al. Eur J Hum Genet. 2023 Nov;31(11):1209-1217. doi: 10.1038/s41431-023-01456-z. Epub 2023 Sep 12. Eur J Hum Genet. 2023. PMID: 37697079 Free PMC article. Review.
-
Fidalgo MF, Fonseca CG, Caldas P, Raposo AA, Balboni T, Henao-Mišíková L, Grosso AR, Vasconcelos FF, Franco CA. Fidalgo MF, et al. Life Sci Alliance. 2022 Oct 11;5(12):e202201554. doi: 10.26508/lsa.202201554. Life Sci Alliance. 2022. PMID: 36220570 Free PMC article.
-
Elavl1 is dispensable for appendicular skeletal development.
Parsha R, Kota SK. Parsha R, et al. bioRxiv [Preprint]. 2024 Sep 24:2024.09.23.614008. doi: 10.1101/2024.09.23.614008. bioRxiv. 2024. PMID: 39386561 Free PMC article. Preprint.
-
Establishing and maintaining fertility: the importance of cell cycle arrest.
Frost ER, Taylor G, Baker MA, Lovell-Badge R, Sutherland JM. Frost ER, et al. Genes Dev. 2021 May 1;35(9-10):619-634. doi: 10.1101/gad.348151.120. Epub 2021 Apr 22. Genes Dev. 2021. PMID: 33888561 Free PMC article. Review.
References
-
- Pepling ME (2006) From primordial germ cell to primordial follicle: mammalian female germ cell development. Genesis 44: 622–632 - PubMed
-
- Bowles J, Knight D, Smith C, Wilhelm D, Richman J, Mamiya S, Yashiro K, Chawengsaksophak K, Wilson MJ, Rossant J et al (2006) Retinoid signaling determines germ cell fate in mice. Science 312: 596–600 - PubMed
-
- Pepling ME, Spradling AC (2001) Mouse ovarian germ cell cysts undergo programmed breakdown to form primordial follicles. Dev Biol 234: 339–351 - PubMed
-
- Soyal SM, Amleh A, Dean J (2000) FIGalpha, a germ cell‐specific transcription factor required for ovarian follicle formation. Development 127: 4645–4654 - PubMed
Publication types
MeSH terms
Substances
Grants and funding
- 17K07442/Grant-in-Aid for Scientific Research (C) from JSPS/International
- 26251025/Grant-in-Aid for Scientific Research (A) from JSPS/International
- 26114512/Grant-in-Aid for Innovative Areas from MEXT/International
- 16H01259/Grant-in-Aid for Innovative Areas from MEXT/International
- 19H05247/Grant-in-Aid for Innovative Areas from MEXT/International
LinkOut - more resources
Full Text Sources
Medical
Molecular Biology Databases