Genetic strategies for improving crop yields - PubMed
Review
Genetic strategies for improving crop yields
Julia Bailey-Serres et al. Nature. 2019 Nov.
Abstract
The current trajectory for crop yields is insufficient to nourish the world's population by 20501. Greater and more consistent crop production must be achieved against a backdrop of climatic stress that limits yields, owing to shifts in pests and pathogens, precipitation, heat-waves and other weather extremes. Here we consider the potential of plant sciences to address post-Green Revolution challenges in agriculture and explore emerging strategies for enhancing sustainable crop production and resilience in a changing climate. Accelerated crop improvement must leverage naturally evolved traits and transformative engineering driven by mechanistic understanding, to yield the resilient production systems that are needed to ensure future harvests.
Conflict of interest statement
Competing interests The authors declare no competing interests.
Figures
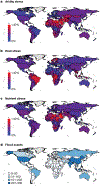
a–c, Maps indicate the yield losses caused by aridity stress averaged from 1950–2000 (a), heat stress averaged from 1994–2010 (b) and nutrient stress in 2009 (c). National data for each crop were previously compiled, and are here averaged and re-plotted using the maps package in R. d, Number of large flood events from 1985 to 2010 by country.
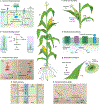
Overview of traits that provide increased resilience and yield in variable environments. a, Pathogen recognition by cell-surface and intracellular receptors (resistance proteins). Manipulation of host cells by pathogen-secreted effectors to promote infection can be recognized by resistance proteins and converted to disease resistance.b, Flooding survival via opposing regulation of gibberellin (GA). Semidwarf 1 (SD1), Snorkel 1 and Snorkel 2 (SK1/2) confer escape by accelerated elongation growth. Submergence 1A (SUB1A) confers tolerance by quiescence of growth. c, Root growth towards moisture involves transcriptional regulators (indol-3-acetic acid inhibitor protein 3 (IAA3) and auxin response factor (ARF7)), and is regulated by the hormones ABA and auxin. d, HKT1 (high-affinity K+ transporter sub-family 1) mediates sodium (Na+) exclusion from leaves. e, In developing seed tissues, catabolism of T6P aids the movement of photo-assimilate carbohydrate (CHO) from leaves to sinks in developing florets. f, Optimizing photosynthetic light harvesting and CO2 fixation by altering photosynthetic protein abundance and minimizing photorespiration. PS, photosystem. g, Dynamic control of stomatal aperture by pairs of epidermal guard cells lessens desiccation. h, Symbiotic plant–microorganism interactions facilitate the uptake of essential nutrients. NH4+, ammonium; PO4−, phosphate; NO3−, nitrate.
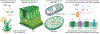
Transgenic manipulations of photosynthetic metabolism that lead to improved photosynthetic efficiency include (1) improving photosynthesis in a dynamic light environment by accelerating recovery from a photoprotected state, by overexpressing enzymes (such as photosystem II subunit S (PSBS) and VDE) that are involved in non-photochemical quenching (NPQ) (the dissipation of excess excitation energy as heat); (2) altering the CO2 response of stomata or the density of stomata on the leaf surface to increase the efficiency of water use,,; (3) increasing the capacity for mesophyll conductance of CO2; (4) improving the energy efficiency of carbon metabolism by altering mitochondrial enzymes; (5) optimizing investment in light collection; (6) increasing electron flow through the photosynthetic electron transport chain; (7) altering Rubisco properties and activation to increase CO2 assimilation,; (8) bypassing photorespiration; and (9) increasing the efficiency of ribulose 1,5-bisphosphate (RuBP) regeneration.
Comment in
-
Innovative plant breeding could deliver crop revolution.
Anders S, Pareek A, Singla-Pareek SL, Gupta KJ, Foyer CH. Anders S, et al. Nature. 2020 Jan;577(7792):622. doi: 10.1038/d41586-020-00186-2. Nature. 2020. PMID: 31992889 No abstract available.
Similar articles
-
Opportunities and challenges for nanotechnology in the agri-tech revolution.
Lowry GV, Avellan A, Gilbertson LM. Lowry GV, et al. Nat Nanotechnol. 2019 Jun;14(6):517-522. doi: 10.1038/s41565-019-0461-7. Epub 2019 Jun 5. Nat Nanotechnol. 2019. PMID: 31168073 Review.
-
Innovative plant breeding could deliver crop revolution.
Anders S, Pareek A, Singla-Pareek SL, Gupta KJ, Foyer CH. Anders S, et al. Nature. 2020 Jan;577(7792):622. doi: 10.1038/d41586-020-00186-2. Nature. 2020. PMID: 31992889 No abstract available.
-
CRISPR, microbes and more are joining the war against crop killers.
Borel B. Borel B. Nature. 2017 Mar 14;543(7645):302-304. doi: 10.1038/543302a. Nature. 2017. PMID: 28300126 No abstract available.
-
Understanding plant-soil interactions underpins enhanced sustainability of crop production.
Wang X, Cheng L, Xiong C, Whalley WR, Miller AJ, Rengel Z, Zhang F, Shen J. Wang X, et al. Trends Plant Sci. 2024 Nov;29(11):1181-1190. doi: 10.1016/j.tplants.2024.05.008. Epub 2024 Jun 18. Trends Plant Sci. 2024. PMID: 38897884 Review.
-
Closing Yield Gaps: How Sustainable Can We Be?
Pradhan P, Fischer G, van Velthuizen H, Reusser DE, Kropp JP. Pradhan P, et al. PLoS One. 2015 Jun 17;10(6):e0129487. doi: 10.1371/journal.pone.0129487. eCollection 2015. PLoS One. 2015. PMID: 26083456 Free PMC article.
Cited by
-
Satyakam, Zinta G, Singh RK, Kumar R. Satyakam, et al. Front Genet. 2022 Aug 25;13:909007. doi: 10.3389/fgene.2022.909007. eCollection 2022. Front Genet. 2022. PMID: 36092945 Free PMC article. Review.
-
Recent advances in understanding and improving photosynthesis.
Perera-Castro AV, Flexas J. Perera-Castro AV, et al. Fac Rev. 2020 Nov 6;9:5. doi: 10.12703/b/9-5. eCollection 2020. Fac Rev. 2020. PMID: 33659937 Free PMC article. Review.
-
Abdullah M, Ahmad F, Zang Y, Jin S, Ahmed S, Li J, Islam F, Ahmad M, Zhang Y, Hu Y, Guan X, Zhang T. Abdullah M, et al. Plant Physiol. 2023 Jan 2;191(1):772-788. doi: 10.1093/plphys/kiac511. Plant Physiol. 2023. PMID: 36342207 Free PMC article.
-
Napier JD, Heckman RW, Juenger TE. Napier JD, et al. Plant Cell. 2023 Jan 2;35(1):109-124. doi: 10.1093/plcell/koac322. Plant Cell. 2023. PMID: 36342220 Free PMC article. Review.
-
Agriculture and food security under a changing climate: An underestimated challenge.
Seppelt R, Klotz S, Peiter E, Volk M. Seppelt R, et al. iScience. 2022 Nov 10;25(12):105551. doi: 10.1016/j.isci.2022.105551. eCollection 2022 Dec 22. iScience. 2022. PMID: 36458255 Free PMC article. Review.
References
-
- Duke SO Perspectives on transgenic, herbicide-resistant crops in the United States almost 20 years after introduction. Pest Manag. Sci 71, 652–657 (2015). - PubMed
-
- Tabashnik BE, Brévault T & Carrière Y Insect resistance to Bt crops: lessons from the first billion acres. Nat. Biotechnol 31, 510–521 (2013). - PubMed
-
- Fitch MMM, Manshardt RM, Gonsalves D, Slightom JL & Sanford JC Virus resistant papaya plants derived from tissues bombarded with the coat protein gene of papaya ringspot virus. Bio/Technology 10, 1466–1472 (1992).
Publication types
MeSH terms
Substances
LinkOut - more resources
Full Text Sources
Other Literature Sources