Epitope-specific airway-resident CD4+ T cell dynamics during experimental human RSV infection - PubMed
- ️Wed Jan 01 2020
Clinical Trial
. 2020 Jan 2;130(1):523-538.
doi: 10.1172/JCI131696.
Agnieszka Jozwik 1 , Stephanie Ascough 2 , Seng Kuong Ung 2 , Suzanna Paterson 2 , Mohini Kalyan 2 , Zoe Gardener 2 , Emma Bergstrom 2 , Satwik Kar 2 , Maximillian S Habibi 1 , Allan Paras 1 , Jie Zhu 1 , Mirae Park 1 , Jaideep Dhariwal 1 , Mark Almond 1 , Ernie Hc Wong 1 , Annemarie Sykes 1 , Jerico Del Rosario 1 , Maria-Belen Trujillo-Torralbo 1 , Patrick Mallia 1 , John Sidney 3 , Bjoern Peters 3 , Onn Min Kon 1 , Alessandro Sette 3 4 , Sebastian L Johnston 1 , Peter J Openshaw 1 , Christopher Chiu 2
Affiliations
- PMID: 31815739
- PMCID: PMC6934186
- DOI: 10.1172/JCI131696
Clinical Trial
Epitope-specific airway-resident CD4+ T cell dynamics during experimental human RSV infection
Aleks Guvenel et al. J Clin Invest. 2020.
Abstract
BACKGROUNDRespiratory syncytial virus (RSV) is an important cause of acute pulmonary disease and one of the last remaining major infections of childhood for which there is no vaccine. CD4+ T cells play a key role in antiviral immunity, but they have been little studied in the human lung.METHODSHealthy adult volunteers were inoculated i.n. with RSV A Memphis 37. CD4+ T cells in blood and the lower airway were analyzed by flow cytometry and immunohistochemistry. Bronchial soluble mediators were measured using quantitative PCR and MesoScale Discovery. Epitope mapping was performed by IFN-γ ELISpot screening, confirmed by in vitro MHC binding.RESULTSActivated CD4+ T cell frequencies in bronchoalveolar lavage correlated strongly with local C-X-C motif chemokine 10 levels. Thirty-nine epitopes were identified, predominantly toward the 3' end of the viral genome. Five novel MHC II tetramers were made using an immunodominant EFYQSTCSAVSKGYL (F-EFY) epitope restricted to HLA-DR4, -DR9, and -DR11 (combined allelic frequency: 15% in Europeans) and G-DDF restricted to HLA-DPA1*01:03/DPB1*02:01 and -DPA1*01:03/DPB1*04:01 (allelic frequency: 55%). Tetramer labeling revealed enrichment of resident memory CD4+ T (Trm) cells in the lower airway; these Trm cells displayed progressive differentiation, downregulation of costimulatory molecules, and elevated CXCR3 expression as infection evolved.CONCLUSIONSHuman infection challenge provides a unique opportunity to study the breadth of specificity and dynamics of RSV-specific T-cell responses in the target organ, allowing the precise investigation of Trm recognizing novel viral antigens over time. The new tools that we describe enable precise tracking of RSV-specific CD4+ cells, potentially accelerating the development of effective vaccines.TRIAL REGISTRATIONClinicalTrials.gov NCT02755948.FUNDINGMedical Research Council, Wellcome Trust, National Institute for Health Research.
Keywords: Adaptive immunity; Immunology; Infectious disease; T cells.
Conflict of interest statement
Conflict of interest: The authors have declared that no conflict of interest exists.
Figures
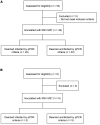
(A) Healthy adult volunteers (n = 49) were enrolled and inoculated with RSV M37 for polyclonal CD4+ T cell analysis and epitope discovery. (B) A second cohort (n = 8) was enrolled for tetramer analysis of RSV-specific CD4+ T cells.
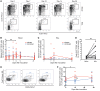
(A) Whole blood (n = 49) and BAL (n = 24) samples were stained with anti-CD3, -CD4, -CD8, -CD38, and –Ki-67 for analysis by flow cytometry. Plots are gated on CD3+CD4+ lymphocytes. One representative infected subject is shown for blood (upper panels) and BAL (lower panels). Median and individual data points of Ki-67+CD38+CD4+ T cells in the (B) blood and (C) BAL of infected (PCR+, red) or uninfected (PCR–, blue) volunteers are shown. Tests of the 5 a priori hypotheses were conducted by Wilcoxon’s signed-rank test with Bonferroni-adjusted α levels of 0.01 (**P < 0.001). (D) Frequencies of Ki-67+CD38+ cells on day 10 after infection are compared between paired blood and BAL samples in infected individuals (n = 12). Tests of the 5 a priori hypotheses were conducted by Wilcoxon’s signed-rank test with Bonferroni-adjusted α levels of 0.01 with no statistically significant differences seen. (E) Whole blood and BAL samples were stained with anti-CD3, -CD4, -FoxP3, and -CD25. One representative infected BAL sample is shown gated on CD3+CD4+ lymphocytes. (F) Mean and individual data points of FoxP3+CD25+CD4+ T cells in the blood and BAL of infected (PCR+, red circles) or uninfected (PCR–, blue squares) volunteers are shown. P values for Wilcoxon’s signed-rank (intragroup) and Mann-Whitney tests (intergroup) are shown. *P < 0.05.
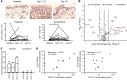
(A) CD4+ cells (brown) were identified in bronchial biopsies (n = 12) by immunohistochemistry and enumerated in infected individuals. Scale bars: 20 μm. Individual data points are presented as number of positive cells per square millimeter of subepithelium or per 0.1 mm2 of epithelium. *P < 0.05 by Wilcoxon’s signed-rank test. (B) Differential cytokine and chemokine gene expression in bronchial brushings 7 days after infection compared with preinfection was analyzed by RT2 Profiler qPCR array. Greater than 2-fold upregulated (red) and downregulated (green) genes are shown. (C) Mean ± SEM gene expression levels of CXCL9, CXCL10, and CXCL11 are shown in infected (red) and uninfected (green) individuals. (D) Spearman’s correlations between bronchial mucosal lining fluid CXCL10 or CXCL11 concentrations and the frequency of Ki-67+CD38+CD4+ T cells in BAL are shown.
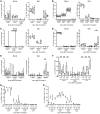
Whole blood/PBMCs (n = 10) and BAL (n = 5) from individuals infected with RSV were costained with anti-CD3, -CD4, and phenotypic markers and then analyzed by flow cytometry. (A) CD69 and CD103 as canonical markers of resident memory T cells are shown in blood and BAL from infected volunteers. Mean ± SEM frequencies are shown. (B) Memory markers CD45RA and CCR7, (C) costimulatory markers CD27 and CD28, (D) homing markers CCR5 and CD62L, and (E) cytotoxicity markers perforin and granzyme B are shown in blood and BAL. In CD69+ (Trm) and CD69– (non-Trm) subsets from BAL, frequencies of (F) CCR5 and CD62L, (G) CD45RA and CCR7, and (H) CD27 and CD28-expressing CD4+ T cell are shown. P values for paired t test are shown. **P < 0.01.
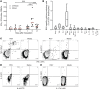
(A) PBMCs from RSV-challenged subjects (n = 18) were assayed by IFN-γ ELISpot stimulated with whole RSV. Median and individual data points are shown with P values for 2-tailed Mann-Whitney test comparing infected and uninfected groups, and 2-tailed Wilcoxon’s matched-pairs tests comparing time points. ***P < 0.001. (B) Median and IQR of T helper cell subsets expressing different cytokines are shown. (C–E) PBMCs from infected subjects (n = 10) on day 10 after infection were cultured in vitro with live RSV or media for 24 hours and Brefeldin A added 4 hours before staining with fixable viability dye and with antibodies against CD3, CD4, and (C) IFN-γ, IL-2, and TNF; (D) IL-4, IL-5; or (E) IL-17A and IL-17F. Representative FACS plots from a single participant are shown.
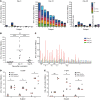
Fresh PBMCs from individuals infected with RSV (n = 10) were assayed by IFN-γ ELISpot using overlapping peptides covering the RSV proteome. (A) ELISpot responses to peptide pools on days 0, 10, and 28 after infection are arranged according to the originating protein. (B) Total ELISpot responses to peptide pools on days 0, 10, and 28 after infection are shown. Median and individual counts are shown with P values for 2-tailed Wilcoxon’s matched-pairs tests comparing time points. (C) Median and IQR ELISpot responses to each peptide pool are shown in RSV genome order. (D) Median and IQR ELISpot responses to G-DDF (n = 3) and (E) F-EFY (n = 3) peptides are shown. P values are for 2-tailed Wilcoxon’s matched-pairs tests. *P < 0.05, **P < 0.01, ***P < 0.001.
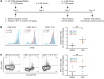
(A) CFSE-stained PBMCs from uninfected HLA-matched donors were cultured with F-EFY, G-DDF, or DMSO and supplemented with IL-2 and IL-7. (B) CFSE dilution was analyzed by flow cytometry (n = 9). PBMCs from 6 representative donors expressing differing HLA alleles are shown. Blue histograms show cultures with peptide epitopes and red show DMSO negative controls. Median and individual data points of frequencies of CFSElo CD4+ T cells after stimulation are plotted. (C) PBMCs (n = 9) were restimulated after 10 days with peptide epitopes and assayed by intracellular staining for IFN-γ and flow cytometry. Medians and individual data points of IFN-γ+CD4+ T cell frequencies are plotted. Two-tailed Mann-Whitney test was used to compare RSV- and DMSO-only responses (**P < 0.01).
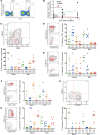
PBMCs from DPA1*01:03/DPB1*04:01–expressing individuals infected with RSV (n = 5) before infection and on day 10 were stained using DPB1*04:01/G-DDF tetramer and analyzed by flow cytometry. (A) FACS plots from 1 representative donor are shown, gated on CD3+ lymphocytes. (B) Cumulative data from blood (n = 11) and BAL (n = 7) are shown with P values for 2-tailed Mann-Whitney test comparing infected and uninfected groups, and 2-tailed Wilcoxon’s matched-pairs tests comparing time points. *P < 0.05, **P < 0.01, ***P < 0.001. PBMCs and BAL cells were costained with phenotypic markers of (C) T cell resident memory (CD69 and CD103), (D) proliferation/activation (Ki-67 and CD38), (E) memory subsets (CD45RA and CCR7), (F) costimulation (CD27 and CD28), homing receptors (G) CCR5 and CD62L and (H) CXCR3 and CCR4. FACS plots from 1 representative donor are shown, gated on CD3+CD4+ T lymphocytes. Red dots represent tetramer+ cells, and gray contours show total CD4+ T cells. The median frequencies of each subset are shown. Where not indicated with asterisks, differences were not statistically significant.
Similar articles
-
Characterization of respiratory syncytial virus M- and M2-specific CD4 T cells in a murine model.
Liu J, Ruckwardt TJ, Chen M, Johnson TR, Graham BS. Liu J, et al. J Virol. 2009 May;83(10):4934-41. doi: 10.1128/JVI.02140-08. Epub 2009 Mar 4. J Virol. 2009. PMID: 19264776 Free PMC article.
-
de Waal L, Yüksel S, Brandenburg AH, Langedijk JP, Sintnicolaas K, Verjans GM, Osterhaus AD, de Swart RL. de Waal L, et al. J Virol. 2004 Feb;78(4):1775-81. doi: 10.1128/jvi.78.4.1775-1781.2004. J Virol. 2004. PMID: 14747542 Free PMC article.
-
Determining the breadth of the respiratory syncytial virus-specific T cell response.
McDermott DS, Knudson CJ, Varga SM. McDermott DS, et al. J Virol. 2014 Mar;88(6):3135-43. doi: 10.1128/JVI.02139-13. Epub 2013 Dec 26. J Virol. 2014. PMID: 24371055 Free PMC article.
-
The CD4 T cell response to respiratory syncytial virus infection.
Christiaansen AF, Knudson CJ, Weiss KA, Varga SM. Christiaansen AF, et al. Immunol Res. 2014 Aug;59(1-3):109-17. doi: 10.1007/s12026-014-8540-1. Immunol Res. 2014. PMID: 24838148 Review.
Cited by
-
Zhang M, Li N, He Y, Shi T, Jie Z. Zhang M, et al. Front Immunol. 2022 Aug 12;13:943331. doi: 10.3389/fimmu.2022.943331. eCollection 2022. Front Immunol. 2022. PMID: 36032142 Free PMC article. Review.
-
Age-dependent nasal immune responses in non-hospitalized bronchiolitis children.
Cortegano I, Rodríguez M, Hernángómez S, Arrabal A, Garcia-Vao C, Rodríguez J, Fernández S, Díaz J, de la Rosa B, Solís B, Arribas C, Garrido F, Zaballos A, Roa S, López V, Gaspar ML, de Andrés B. Cortegano I, et al. Front Immunol. 2022 Dec 6;13:1011607. doi: 10.3389/fimmu.2022.1011607. eCollection 2022. Front Immunol. 2022. PMID: 36561744 Free PMC article.
-
Humphries DC, O'Connor RA, Larocque D, Chabaud-Riou M, Dhaliwal K, Pavot V. Humphries DC, et al. Front Immunol. 2021 Sep 17;12:738955. doi: 10.3389/fimmu.2021.738955. eCollection 2021. Front Immunol. 2021. PMID: 34603321 Free PMC article. Review.
-
Schmidt A, Lapuente D. Schmidt A, et al. Viruses. 2021 Jan 28;13(2):199. doi: 10.3390/v13020199. Viruses. 2021. PMID: 33525620 Free PMC article. Review.
-
Ferguson M, Schwarz TF, Núñez SA, Rodríguez-García J, Mital M, Zala C, Schmitt B, Toursarkissian N, Mazarro DO, Großkopf J, Voors-Pette C, Mehta H, Hailemariam HA, de Heusch M, Salaun B, Damaso S, David MP, Descamps D, Hill J, Vandermeulen C, Hulstrøm V; RSV OA=ADJ-018 Study Group. Ferguson M, et al. Clin Infect Dis. 2024 Oct 15;79(4):1074-1084. doi: 10.1093/cid/ciae364. Clin Infect Dis. 2024. PMID: 39099093 Free PMC article. Clinical Trial.
References
Publication types
MeSH terms
Substances
Grants and funding
LinkOut - more resources
Full Text Sources
Medical
Research Materials