Cell-line specific RNA editing patterns in Trypanosoma brucei suggest a unique mechanism to generate protein variation in a system intolerant to genetic mutations - PubMed
- ️Wed Jan 01 2020
Cell-line specific RNA editing patterns in Trypanosoma brucei suggest a unique mechanism to generate protein variation in a system intolerant to genetic mutations
Laura E Kirby et al. Nucleic Acids Res. 2020.
Abstract
Trypanosoma brucei possesses a highly complex RNA editing system that uses guide RNAs to direct the insertion and deletion of uridines in mitochondrial mRNAs. These changes extensively alter the target mRNAs and can more than double them in length. Recently, analyses showed that several of the edited genes possess the capacity to encode two different protein products. The overlapped reading frames can be accessed through alternative RNA editing that shifts the translated reading frame. In this study, we analyzed the editing patterns of three putative dual-coding genes, ribosomal protein S12 (RPS12), the 5' editing domain of NADH dehydrogenase subunit 7 (ND7 5'), and C-rich region 3 (CR3). We found evidence that alternatively 5'-edited ND7 5' and CR3 transcripts are present in the transcriptome, providing evidence for the use of dual ORFs in these transcripts. Moreover, we found that CR3 has a complex set of editing pathways that vary substantially between cell lines. These findings suggest that alternative editing can work to introduce genetic variation in a system that selects against nucleotide mutations.
© The Author(s) 2019. Published by Oxford University Press on behalf of Nucleic Acids Research.
Figures
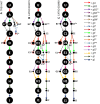
Observed RPS12 editing pathways in the TREU 667 cell line (A) and the EATRO 164 cell line grown in SDM79 (B) and SDM80 (C). U = unedited transcripts. Dot sizes are proportional to the percent of block level edited transcripts using the gRNA indicated. Colored arrows indicate the gRNA population used. Dashed arrows with closed heads represent gRNA populations used in only one cell line (superscript ‘e’ or ‘t’). gRNA names: superscript ‘p’ represent promiscuous gRNAs that can anchor and edit more than one editing block; superscript ‘FS’ indicate gRNAs that introduce a frameshift; * indicates gRNAs that introduce a minor aa change. End point mRNAs with no evidence of further editing are represented by dots enclosed by a red box and the mRNAs are named with a superscript ‘x’. gFp is a promiscuous gRNA that edits both in the D and F editing block of RPS12. Arrows with a boxed ‘m’ represent a gRNA that has mis-anchored.
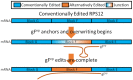
Model of how gFep can overwrite fully edited transcripts to generate observed mRNA transcript sequence patterns. mRNAs are represented by rectangles, with editing blocks labeled. gFep is shown as an orange line. Blue blocks are conventionally edited, orange blocks are alternatively edited, and blocks fading from blue to orange are in an incompletely edited junction state. Overwriting of block F resulting in the decrease in editing efficiency observed at block F as compared to block G (Table 2).
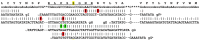
Regions with poor gRNA coverage and functionally conserved residues in RPS12. Functionally important aa residues are underlined (72). Pipes (|) indicate Watson/Crick base pairs and colons (:) indicate G/U base pairs. Red highlighted hashtags (#) indicated gaps or mismatches, green highlighted hashtags indicate C:A base pairs. The introduced substitution mutation introduced by use of the gGe gRNA is highlighted in yellow (S>P).
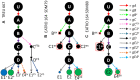
Observed ND7 5′ editing pathways in the TREU 667 cell line (A) and the EATRO 164 cell line grown in SDM79 (B) and SDM80 (C). U = unedited transcripts. For arrow, gRNA and mRNA naming descriptors see Figure 1. + indicates that more than one mRNA form was condensed into this circle to simplify the figure (See Supplementary Figure S4). Condensed forms encode largely the same amino acid sequence with only small variants. Terminal dots are colored blue for reading frame 1, magenta for reading frame 2, or green for reading frame 3. Boxed green dots have no in frame AUG start codon but are translatable into reading frame 3 with the use of an alternative start codon (UUG).

Regions with poor gRNA coverage and functionally conserved residues in ND7′5′. Functionally important histidine residues are underlined (51). Pipes indicate Watson/Crick base pairs and colons indicate G/U base pairs. Red highlighted hashtags (#) indicate gaps or mismatches, green highlighted hashtags indicate C/A base pairs.
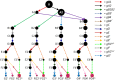
Observed CR3 editing pathways in the TREU 667 cell line. U = unedited transcripts. For arrow and gRNA naming descriptors see Figure 1. gRNAs with prime designations indicate extensively overlapped gRNAs both needed to cover a single editing block. Terminal dots are colored blue for reading frame 1 or magenta for reading frame 2. Boxed magenta dots have no functional start codon but are translatable into reading frame 2 with the use of an alternative start codon (UUG).
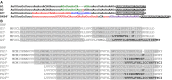
(A) Four different 3′ end sequences found in the TREU 667 transcriptome for the CR3 transcript. U-residues inserted by editing are indicated by lowercase; different sequences created by the different gRNAs are highlighted in RED. The additional 3 U-residues inserted by gA2 are indicated by dotted underline. Thick underline sequence indicates the anchor binding site (ABS) for the initiating gRNAs (gA1 and gA2). Green = ABS for gB1B2; Blue = ABS for gB3t; Purple = ABS for gB4. (B) CR3 protein sequences for both reading frame 1 (ORF) and reading frame 2 (ARF). Bolded amino acids show sequence variants and shaded sequence shows position of predicted transmembrane domains (73). mRNAs with no functional AUG are named with a superscript ‘x’.
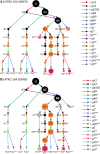
Observed CR3 editing pathways in the EATRO 164 cell line grown in SDM79 (A) and SDM80 (B). U = unedited transcripts. For arrow and gRNA naming descriptors see Figure 1. Orange dots indicate edited sequence unique to the EATRO 164 cell line. Green dots indicate edited sequence unique to EATRO cells grown in SDM80 medium. Terminal dots are colored blue for reading frame 1 or magenta for reading frame 2. Boxed magenta dots have no functional start codon but are translatable into reading frame 2 with the use of an alternative start codon (UUG). + indicates that more than one mRNA form was condensed into this circle to simplify the figure (see Supplementary Figure S6). Condensed forms encode largely the same amino acid sequence with only small variants.
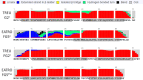
Predicted secondary structures of most abundant CR3 predicted proteins. Secondary structure predictions were generated by RaptorX (74–76). Shaded regions indicate predicted transmembrane alpha helices predicted by Phobius (73,77).
Similar articles
-
Aphasizheva I, Maslov DA, Aphasizhev R. Aphasizheva I, et al. RNA Biol. 2013 Nov;10(11):1679-88. doi: 10.4161/rna.26733. Epub 2013 Oct 14. RNA Biol. 2013. PMID: 24270388 Free PMC article.
-
Souza AE, Shu HH, Read LK, Myler PJ, Stuart KD. Souza AE, et al. Mol Cell Biol. 1993 Nov;13(11):6832-40. doi: 10.1128/mcb.13.11.6832-6840.1993. Mol Cell Biol. 1993. PMID: 8413276 Free PMC article.
-
Ochsenreiter T, Cipriano M, Hajduk SL. Ochsenreiter T, et al. PLoS One. 2008 Feb 13;3(2):e1566. doi: 10.1371/journal.pone.0001566. PLoS One. 2008. PMID: 18270563 Free PMC article.
-
The RNA editing process in Trypanosoma brucei.
Stuart K. Stuart K. Semin Cell Biol. 1993 Aug;4(4):251-60. doi: 10.1006/scel.1993.1030. Semin Cell Biol. 1993. PMID: 8241468 Review.
-
U-Insertion/Deletion mRNA-Editing Holoenzyme: Definition in Sight.
Aphasizheva I, Aphasizhev R. Aphasizheva I, et al. Trends Parasitol. 2016 Feb;32(2):144-156. doi: 10.1016/j.pt.2015.10.004. Epub 2015 Nov 10. Trends Parasitol. 2016. PMID: 26572691 Free PMC article. Review.
Cited by
-
Smith JT Jr, Doleželová E, Tylec B, Bard JE, Chen R, Sun Y, Zíková A, Read LK. Smith JT Jr, et al. Nucleic Acids Res. 2020 Sep 4;48(15):8704-8723. doi: 10.1093/nar/gkaa641. Nucleic Acids Res. 2020. PMID: 32738044 Free PMC article.
-
RESC14 and RESC8 cooperate to mediate RESC function and dynamics during trypanosome RNA editing.
Wackowski K, Zhu X, Shen S, Zhang M, Qu J, Read LK. Wackowski K, et al. Nucleic Acids Res. 2024 Sep 9;52(16):9867-9885. doi: 10.1093/nar/gkae561. Nucleic Acids Res. 2024. PMID: 38967000 Free PMC article.
-
RPL19 Is a Prognostic Biomarker and Promotes Tumor Progression in Hepatocellular Carcinoma.
Rao B, Li J, Ren T, Yang J, Zhang G, Liu L, Wang H, Huang M, Ren Z, Yu Z. Rao B, et al. Front Cell Dev Biol. 2021 Jul 19;9:686547. doi: 10.3389/fcell.2021.686547. eCollection 2021. Front Cell Dev Biol. 2021. PMID: 34350180 Free PMC article.
-
Gerasimov ES, Gasparyan AA, Afonin DA, Zimmer SL, Kraeva N, Lukeš J, Yurchenko V, Kolesnikov A. Gerasimov ES, et al. Nucleic Acids Res. 2021 Apr 6;49(6):3354-3370. doi: 10.1093/nar/gkab114. Nucleic Acids Res. 2021. PMID: 33660779 Free PMC article.
-
Gerasimov ES, Ramirez-Barrios R, Yurchenko V, Zimmer SL. Gerasimov ES, et al. RNA. 2022 Jul;28(7):993-1012. doi: 10.1261/rna.079088.121. Epub 2022 Apr 25. RNA. 2022. PMID: 35470233 Free PMC article.
References
-
- Shapiro T.A., Englund P.T.. The structure and replication of kinetoplast DNA. Annu. Rev. Microbiol. 1995; 49:117–143. - PubMed
-
- Priest J.W., Hajduk S.L.. Developmental regulation of mitochondrial biogenesis in Trypanosoma brucei. J. Bioenerg. Biomembr. 1994; 26:179–191. - PubMed
-
- Blum B., Bakalara N., Simpson L.. A model for RNA editing in kinetoplastid mitochondria: ‘Guide’ RNA molecules transcribed from maxicircle DNA provide the edited information. Cell. 1990; 60:189–198. - PubMed
Publication types
MeSH terms
Substances
LinkOut - more resources
Full Text Sources
Miscellaneous