Parietal Cortex Regulates Visual Salience and Salience-Driven Behavior - PubMed
- ️Wed Jan 01 2020
Parietal Cortex Regulates Visual Salience and Salience-Driven Behavior
Xiaomo Chen et al. Neuron. 2020.
Abstract
Unique stimuli stand out. Despite an abundance of competing sensory stimuli, the detection of the most salient ones occurs without effort, and that detection contributes to the guidance of adaptive behavior. Neurons sensitive to the salience of visual stimuli are widespread throughout the primate visual system and are thought to shape the selection of visual targets. However, a neural source of salience remains elusive. In an attempt to identify a source of visual salience, we reversibly inactivated parietal cortex and simultaneously recorded salience signals in prefrontal cortex. Inactivation of parietal cortex not only caused pronounced and selective reductions of salience signals in prefrontal cortex but also diminished the influence of salience on visually guided behavior. These observations demonstrate a causal role of parietal cortex in regulating salience signals within the brain and in controlling salience-driven behavior.
Keywords: Attention; choice; eye movement; free-viewing; frontal cortex; receptive field; spatial neglect.
Copyright © 2020 Elsevier Inc. All rights reserved.
Conflict of interest statement
Declaration of Interests The authors declare no competing interests.
Figures
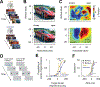
(A) Free-viewing task. Images presented to the monkeys included real-word photographs, paintings, cartoons, and abstract patterns. Identical images were presented during both control (top, gold shading in IPS) and inactivation blocks (bottom, blue shading in IPS). (B) Example image presented to one monkey during a control (top) and inactivation block (bottom). Circles indicate regions of fixation and the lines indicate saccades. The origin of the coordinate system indicates the initial fixation of the monkey at the onset of the image. (C) Change in fixation densities across the population of images for Monkey J (top) and Monkey Q (bottom). The left part of the color maps corresponds to the visual field contralateral to the inactivated PPC, in head-centered coordinates. (D) Double-target, choice task. Two targets were presented at varying temporal onset asynchronies; contralateral targets could trail (-) or lead (+) ipsilateral targets. (E) Example experimental session for one monkey. Target choice functions during control and during PPC inactivation are plotted in gold and blue, respectively. Positive values denote contralateral leading targets. (F) Distribution of shifts in the PES across all sessions in the two monkeys.
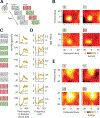
(A) Visual stimuli consisted of a single colored stimulus presented in isolation (Isolated), or among an array (6 ✕ 4) of identically colored stimuli (Unique). (B) Example CRFs of a single FEF neuronal recording mapped with an isolated red or green stimulus (top) and URFs of the same neuronal recording mapped with a unique red or green stimulus (bottom). Responses were normalized across stimulus conditions. Icons above each RF denote stimulus conditions, but not the full array. (C) Spiking responses from an example neuronal recording to isolated and unique stimuli presented inside the CRF/URF (dark gold), shown with responses to single and unique stimuli presented outside of the CRF/URF or to identically colored stimulus arrays (light gold) for two color polarities (left icons). (D) Response spectra of an example FEF LFP recording. Same conventions as in C. (E) High-gamma band CRFs and URFs for an example recording. Responses were normalized across stimulus conditions.
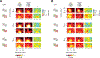
(A) CRFs and URFs for three example neuronal recordings during control (left) and PPC inactivation (middle), shown together with their respective difference maps (inactivation - control) (right). Activity for each recording was normalized across all stimulus and experimental conditions (B) CRFs and URFs for three example LFP recordings (high-gamma band). Same organization and notation as in A.
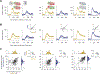
(A) Mean responses of an example neuron to different stimulus conditions during control (gold) and PPC inactivation (blue). Left, responses to UniqueIn (dark) and UniqueOut (light) stimuli. Middle, responses of the same neuron to UniqueIn (dark) and Identical (light). Right, responses of the same neuron to the isolated stimuli presented inside (dark) and outside of the CRF (light). Shading around the response denotes ±SEM. (B) Mean responses for all modulated neurons (n = 193) during control and inactivation blocks for each of the stimulus comparisons (UniqueIn vs. UniqueOut, UniqueIn vs. Identical, IsolatedIn vs. IsolatedOut). Same notation as in A. Gray scatterplots show the reduction in response differences for all recordings (n = 352). (C) Accuracy of classifiers trained on neuronal spiking activity to discriminate between different stimulus conditions during control and PPC inactivation. Left, accuracy of classifiers trained to discriminate between UniqueIn and UniqueOut stimuli. Middle, accuracy of classifiers trained to discriminate between UniqueIn and Identical stimuli. Right, accuracy of classifiers trained to discriminate between Isolated stimuli appearing inside and outside of the CRF. Scatter plots and marginal distributions compare discrimination accuracies across stimulus selective neuronal recordings (n = 193) during control and inactivation. Gray scatterplots show the reduction in enhancement indices during inactivation as a function of enhancement indices measured during control for all recordings (n = 352). Black lines show the linear regression fits.
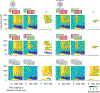
Comparison of average LFP time-frequency power spectrograms during control (left) and PPC inactivation (right) (N=192). The first row compares the spectrograms of responses to UniqueIn and UniqueOut stimuli, and their differences (∆) during control and inactivation. The second row compares responses to UniqueIn and Identical stimuli, and the third row compares responses to Identical stimuli presented inside or outside of the CRF. Difference plots only show time-frequency bins with significant energy differences.
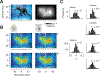
(A) Example image from the free viewing task (top left) and corresponding salience map (top right). (B) Correspondence between salience and fixations made in the example image before and after PPC inactivation. Top row fixations are labelled in eye-centered coordinates as contralaterally (triangles) or ipsilaterally (circles) directed movements. Bottom row shows the same fixations labelled in head-centered coordinates as landing in the contralateral or ipsilateral half of the image. (C) Distribution of changes in fixation-salience map correlation coefficients (rinactivation – rcontrol) across the population of images for the two monkeys. Left histograms show distributions based on coefficients measured from fixations across the full image. Right histograms show distributions based on contralateral fixations, defined in eye-centered (ContraE) or head-centered (ContraH) coordinates.
Similar articles
-
Early involvement of prefrontal cortex in visual bottom-up attention.
Katsuki F, Constantinidis C. Katsuki F, et al. Nat Neurosci. 2012 Jul 22;15(8):1160-6. doi: 10.1038/nn.3164. Nat Neurosci. 2012. PMID: 22820465 Free PMC article.
-
Robinson DL, Goldberg ME. Robinson DL, et al. Fed Proc. 1978 Jul;37(9):2258-61. Fed Proc. 1978. PMID: 95977
-
Salience representation in the parietal and frontal cortex.
Zenon A, Filali N, Duhamel JR, Olivier E. Zenon A, et al. J Cogn Neurosci. 2010 May;22(5):918-30. doi: 10.1162/jocn.2009.21233. J Cogn Neurosci. 2010. PMID: 19366288
-
The role of the parietal cortex in the neural processing of saccadic eye movements.
Bisley JW, Goldberg ME. Bisley JW, et al. Adv Neurol. 2003;93:141-57. Adv Neurol. 2003. PMID: 12894406 Review.
-
The neural instantiation of a priority map.
Bisley JW, Mirpour K. Bisley JW, et al. Curr Opin Psychol. 2019 Oct;29:108-112. doi: 10.1016/j.copsyc.2019.01.002. Epub 2019 Jan 11. Curr Opin Psychol. 2019. PMID: 30731260 Free PMC article. Review.
Cited by
-
Neuroelectrophysiology-compatible electrolytic lesioning.
Bray IE, Clarke SE, Casey KM, Nuyujukian P; Brain Interfacing Laboratory. Bray IE, et al. Elife. 2024 Sep 11;12:RP84385. doi: 10.7554/eLife.84385. Elife. 2024. PMID: 39259198 Free PMC article.
-
Feature-Specific Salience Maps in Human Cortex.
Thayer DD, Sprague TC. Thayer DD, et al. J Neurosci. 2023 Dec 13;43(50):8785-8800. doi: 10.1523/JNEUROSCI.1104-23.2023. J Neurosci. 2023. PMID: 37907257 Free PMC article.
-
Topographical Representation of Saliency in the Human Visual and Temporo-occipital Cortex.
Li KKY, Wong NHL. Li KKY, et al. J Neurosci. 2024 May 8;44(19):e0037242024. doi: 10.1523/JNEUROSCI.0037-24.2024. J Neurosci. 2024. PMID: 38719457 Free PMC article. Review. No abstract available.
-
Neural basis of concurrent deliberation toward a choice and degree of confidence.
Vivar-Lazo M, Fetsch CR. Vivar-Lazo M, et al. bioRxiv [Preprint]. 2024 Sep 27:2024.08.06.606833. doi: 10.1101/2024.08.06.606833. bioRxiv. 2024. PMID: 39149300 Free PMC article. Preprint.
-
Guo Y, Fu J, Hong J, Liu Z, He X. Guo Y, et al. BMJ Open. 2022 Feb 24;12(2):e055848. doi: 10.1136/bmjopen-2021-055848. BMJ Open. 2022. PMID: 35210343 Free PMC article. Clinical Trial.
References
-
- Allman J, Miezin F, and McGuinness E (1985). Stimulus specific responses from beyond the classical receptive field: neurophysiological mechanisms for local-global comparisons in visual neurons. Annu. Rev. Neurosci 8, 407–430. - PubMed
-
- Bichot NP, Schall JD, and Thompson KG (1996). Visual feature selectivity in frontal eye fields induced by experience in mature macaques. Nature 381, 697–699. - PubMed
-
- Borji A, Sihite DN, and Itti L (2013). Quantitative Analysis of Human-Model Agreement in Visual Saliency Modeling: A Comparative Study. IEEE Transactions on Image Processing 22, 55–69. - PubMed