Multiple network properties overcome random connectivity to enable stereotypic sensory responses - PubMed
- ️Wed Jan 01 2020
Multiple network properties overcome random connectivity to enable stereotypic sensory responses
Aarush Mohit Mittal et al. Nat Commun. 2020.
Abstract
Connections between neuronal populations may be genetically hardwired or random. In the insect olfactory system, projection neurons of the antennal lobe connect randomly to Kenyon cells of the mushroom body. Consequently, while the odor responses of the projection neurons are stereotyped across individuals, the responses of the Kenyon cells are variable. Surprisingly, downstream of Kenyon cells, mushroom body output neurons show stereotypy in their responses. We found that the stereotypy is enabled by the convergence of inputs from many Kenyon cells onto an output neuron, and does not require learning. The stereotypy emerges in the total response of the Kenyon cell population using multiple odor-specific features of the projection neuron responses, benefits from the nonlinearity in the transfer function, depends on the convergence:randomness ratio, and is constrained by sparseness. Together, our results reveal the fundamental mechanisms and constraints with which convergence enables stereotypy in sensory responses despite random connectivity.
Conflict of interest statement
The authors declare no competing interests.
Figures
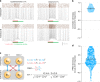
a Raster plots with representative recordings showing responses of the locust bLN1 MBON in two individuals to two odors. Odor was presented for 1 second (red bar) and the spikes in the responses were counted in a 2-s window following odor onset (green bar); scale bar for recordings, 10 mV. In this sample, the responses appear to be stereotypic, with more spikes for octanol 0.1% and fewer spikes for cyclohexanone 0.1% in both the individuals. b Violin plot showing the value of correlation stereotypy in bLN1 responses in the locust dataset. Each point represents the Pearson’s correlation coefficient, calculated using the 6-length vector (responses to 6 odors) for a pair of individuals (n = 15 pairs of individuals). c Schematic description of the PRED metric for stereotypy and comparison with the correlation metric. d PRED stereotypy in bLN1 responses in the locust dataset used in (b). Note the bias towards positive values. Each point represents the pairwise PRED stereotypy value, calculated for a pair of odor responses in a pair of individuals, giving a total of 225 values (15 pairs of odors × 15 pairs of individuals). In b, d black horizontal line represents the mean. Error bars represent s.e.m.
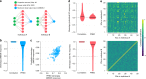
a Schematic representation of the simulated mushroom body networks in two different individuals. Projection neuron (PN, green) to Kenyon cell (KC, pink) connections are random and vary across individuals. The number of KCs connected to the mushroom body output neuron (MBON, blue) was kept same across individuals. b Correlation stereotypy and PRED stereotypy in MBON response in a realistic network with random PN-KC connections across individuals; for correlation, n = 100 points corresponding to different network iterations with different random seeds; for PRED, n = 495000 points (100 iterations × 950 odor pairs from 100 odors). c Scatter plot of correlation stereotypy versus PRED stereotypy in MBON response for the same simulations as in (b) shows that both metrics behave similarly. The PRED stereotypy is averaged over all 4950 odor pairs within a network iteration (n = 100 iterations). d (top) Correlation stereotypy and PRED stereotypy in individual KC response for the same simulations as in (b). Both metrics confirm the absence of stereotypy in individual KCs, n = 100537 KCs that responded to at least one odor in both individuals, out of the 200,000 KCs from 100 network iterations. (bottom) Correlation stereotypy and PRED stereotypy in total KC response for the same simulations as in (b); number of data points same as in (b). e Correlation between the responses of the PNs (bottom) and the KCs (top) in two individuals in a simulation. The correlation is calculated using the 100-length vector (responses to 100 odors) for each pair of PNs (50 in total) or for each pair of KCs among the first 50 KCs (by spatial ordering) that responded to at least one odor in both individuals.
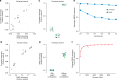
a, b Comparison of PRED stereotypy with correlation stereotypy in the responses of the readout neuron in the simulations of the untrained (a) or trained (b) piriform network using code provided by Schaffer et al.. c, d Stereotypy in the simulations of the untrained piriform network, with and without the weight normalization step calculated using both correlation (c) and PRED (d) metrics. The three different colors represent simulations with different levels of overlap among the olfactory bulb responses to odors as described by Schaffer et al.: 70% overlap (green), 30% overlap (blue) and 0% overlap (gray). a–d have 18 data points each (6 iterations × 3 sets of odors). e Stereotypy in MBON response reduces with the learning rate, as seen in simulations with 100 odors and 2 individuals. Learning of an odor resulted in either increasing or decreasing the number of synapses between the MBON and the KCs activated by that odor. Half of the odors, selected randomly for each individual, were learnt sequentially, and each learning event resulted in increase or decrease in synapses with equal probability. When the synapses were increased, of all the KCs that were activated by the learned odor and were not connected to the MBON, a certain fraction (indicated by the learning rate) were connected to the MBON. When the synapses were reduced, of all the KCs that were activated by the learned odor and were connected to the MBON, the same fraction were disconnected from the MBON. The MBON responses for both individuals were recalculated after modifying the synapses, n = 100 iterations. Error bars represent s.e.m. f Analytical model proved the existence of stereotypy in absence of learning. Plot of stereotypy versus the number of KCs suggested a dependence of stereotypy on the size of the network, as later seen in the simulations (compare to Fig. 6d). Each value is calculated using the formulae derived in the analytical model (see Supplementary equations). Arrow indicates the default value (matching the Drosophila system).
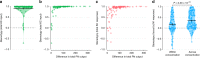
a Stereotypy in total KC input in the simulations. PRED metric was used for calculation of stereotypy in these and all subsequent analyses. b, c Scatter plots of stereotypy in total KC input (b) or total KC response (c) versus the difference in total PN outputs between the two odors. a–c Each point represents a network iteration (n = 100). d Stereotypy in locust bLN1 responses is more for the across-concentration group than the within-concentration group. The within-concentration group has n = 90 stereotypy values (15 pairs of individuals × 3 pairs of odors × 2 concentration levels) and the across-concentration group has n = 135 stereotypy values (15 pairs of individuals × 9 pairs of odors such that one odor in the pair is at 0.1% concentration and the other odor is at 10% concentration). In a, d error bars represent s.e.m.
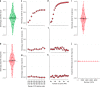
a, b Stereotypy in total KC input (a) and total KC response (b) when the total input drives to both odors are equal. c Stereotypy in total KC response versus the range of PN spiking rates when the range of spiking rates is changed to the indicated values for one odor while it is maintained at 10–30 for the other. d Stereotypy in total KC response versus the number of active PNs when the number is changed to the indicated values for one odor while it is maintained at 25 for the other. e Similar to (c) except that the range of PN spiking rates is changed for both odors. f Similar to (d) except that the number of active PNs is changed for both odors. g, h Plots similar to (c) and (d), respectively, but with the modification that a linear transfer function, yi = mxi − t, is used to generate KC responses from their inputs. Here, yi is the response of the ith KC, xi is the input to the ith KC, t is the threshold, and m is chosen such that the total KC responses are equal to those seen in the default simulations. i Stereotypy in total KC response when the PN responses to the second odor are generated by shuffling the PN labels in the response to the first odor. In this condition, the total PN output, the number of active PNs, and the spiking range of active PNs are identical for the two odors. j Analytical model confirmed that there is no stereotypy, regardless of the number of KCs, when the PN responses to the two odors are shuffled versions of each other. In all panels, n = 100 iterations; error bars represent s.e.m.
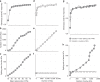
a–c Stereotypy in total KC response (a), average number of active KCs (b), and the average rate of active KCs (c) versus the mean spiking rate of PNs. In this set of simulations, the mean spiking rate of PNs was changed by changing the PN spiking range to the indicated mean ± 10. Note that in these simulations, both the average number of active KCs and their average spiking rate increase with the increase in mean PN spiking rate. d–f Stereotypy in total KC response (d), average number of active KCs (e) and the average rate of active KCs (f) versus the number of KCs in the model, when this number was changed to the indicated value in a separate set of simulations. Note that in these simulations, only the average number of active KCs but not their average spiking rate increases with the number of KCs. g, h Stereotypy in total KC response (g) and the rate of active KCs (h) versus the average number of active KCs in two sets of simulations described in the previous panels. Stereotypy increased equally in both sets of simulations with the number of active KCs and did not depend on the average spiking rate of KCs, which increased in the first set but not in the second set. In all panels, n = 100 iterations; in each iteration, averages were taken over both odors and both individuals. Error bars represent s.e.m.
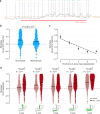
a A representative intracellular recording (gray) from locust bLN1 is shown to illustrate the method of removing spikes that occur at depolarizations below a threshold (dashed line). Discarded spikes are labeled ‘−’ and retained spikes are labeled ‘+’. The depolarization (dark black trace) is extracted by clipping spikes and filtering the recording (50-Hz low-pass). The threshold is set at one s.d. above the mean depolarization. b Stereotypy in locust bLN1 response reduces when we mimic more sparseness by removing spikes below the threshold (1 s.d. above mean depolarization). c Stereotypy continues to reduce as sparseness is further increased by raising the threshold in multiples of s.d. above mean depolarization. In b, c, n = 225 values (15 pairs of odors × 15 pairs of individuals). d Fly KC populations imaged at different lobes of the mushroom body in control flies reveal significant stereotypy. The stereotypy further increases in all lobes as sparseness is reduced by blocking APL output (APL>TNT); n = 315 (control, α- and α′-lobes, from 11 hemispheres from 10 flies), n = 570 (APL>TNT, α- and α′-lobes, from 20 hemispheres from 14 flies), n = 828 (control, β-, β′- and γ-lobes, from 18 hemispheres from 15 flies), n = 1890 (APL>TNT, β-, β′- and γ-lobes, from 36 hemispheres from 24 flies). Error bars represent s.e.m.
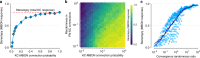
a Stereotypy in MBON response versus the connection probability between KCs and MBON in the model. When connection probability is 1, the MBON stereotypy matches the stereotypy in total KC response (dashed line). Error bars represent s.e.m. b Stereotypy in MBON response as a function of KC-MBON connection probability and the randomness in PN-KC connections (i.e., the fraction of PN-KC synapses whose values are independently set in the two individuals). Simulations were done for 21 values of each parameter in the range 0.01 to 1.0 on a log scale. c Stereotypy in MBON response versus the ratio of convergence (KC-MBON connection probability) to randomness (in PN-KC connections) in the data shown in (b). The dependence of stereotypy (S) on the convergence:randomness ratio (r) is captured well by a fit to the Hill equation, S=r0.650.48+r0.65 (black trace); blue trace shows the average stereotypy of points with the same ratio. In all panels, n = 100 iterations.
Similar articles
-
Presynaptic developmental plasticity allows robust sparse wiring of the Drosophila mushroom body.
Elkahlah NA, Rogow JA, Ahmed M, Clowney EJ. Elkahlah NA, et al. Elife. 2020 Jan 8;9:e52278. doi: 10.7554/eLife.52278. Elife. 2020. PMID: 31913123 Free PMC article.
-
Testing odor response stereotypy in the Drosophila mushroom body.
Murthy M, Fiete I, Laurent G. Murthy M, et al. Neuron. 2008 Sep 25;59(6):1009-23. doi: 10.1016/j.neuron.2008.07.040. Neuron. 2008. PMID: 18817738 Free PMC article.
-
Random convergence of olfactory inputs in the Drosophila mushroom body.
Caron SJ, Ruta V, Abbott LF, Axel R. Caron SJ, et al. Nature. 2013 May 2;497(7447):113-7. doi: 10.1038/nature12063. Epub 2013 Apr 24. Nature. 2013. PMID: 23615618 Free PMC article.
-
Olfactory learning skews mushroom body output pathways to steer behavioral choice in Drosophila.
Owald D, Waddell S. Owald D, et al. Curr Opin Neurobiol. 2015 Dec;35:178-84. doi: 10.1016/j.conb.2015.10.002. Epub 2015 Nov 3. Curr Opin Neurobiol. 2015. PMID: 26496148 Free PMC article. Review.
-
Mushroom body memoir: from maps to models.
Heisenberg M. Heisenberg M. Nat Rev Neurosci. 2003 Apr;4(4):266-75. doi: 10.1038/nrn1074. Nat Rev Neurosci. 2003. PMID: 12671643 Review. No abstract available.
Cited by
-
Information flow, cell types and stereotypy in a full olfactory connectome.
Schlegel P, Bates AS, Stürner T, Jagannathan SR, Drummond N, Hsu J, Serratosa Capdevila L, Javier A, Marin EC, Barth-Maron A, Tamimi IF, Li F, Rubin GM, Plaza SM, Costa M, Jefferis GSXE. Schlegel P, et al. Elife. 2021 May 25;10:e66018. doi: 10.7554/eLife.66018. Elife. 2021. PMID: 34032214 Free PMC article.
-
Kymre JH, Liu X, Ian E, Berge CN, Wang G, Berg BG, Zhao X, Chu X. Kymre JH, et al. Elife. 2021 May 14;10:e65683. doi: 10.7554/eLife.65683. Elife. 2021. PMID: 33988500 Free PMC article.
-
Bansal R, Nagel M, Stopkova R, Sofer Y, Kimchi T, Stopka P, Spehr M, Ben-Shaul Y. Bansal R, et al. BMC Biol. 2021 Jun 28;19(1):133. doi: 10.1186/s12915-021-01064-7. BMC Biol. 2021. PMID: 34182994 Free PMC article.
-
Efficient information coding and degeneracy in the nervous system.
Seenivasan P, Narayanan R. Seenivasan P, et al. Curr Opin Neurobiol. 2022 Oct;76:102620. doi: 10.1016/j.conb.2022.102620. Epub 2022 Aug 17. Curr Opin Neurobiol. 2022. PMID: 35985074 Free PMC article. Review.
-
Prisco L, Deimel SH, Yeliseyeva H, Fiala A, Tavosanis G. Prisco L, et al. Elife. 2021 Dec 29;10:e74172. doi: 10.7554/eLife.74172. Elife. 2021. PMID: 34964714 Free PMC article.
References
Publication types
MeSH terms
LinkOut - more resources
Full Text Sources
Molecular Biology Databases